Understanding Binding Kinetics To Optimize Drug Discovery
The success of a drug candidate not only depends on the drug–target binding affinity, but also on the drug’s binding kinetics.
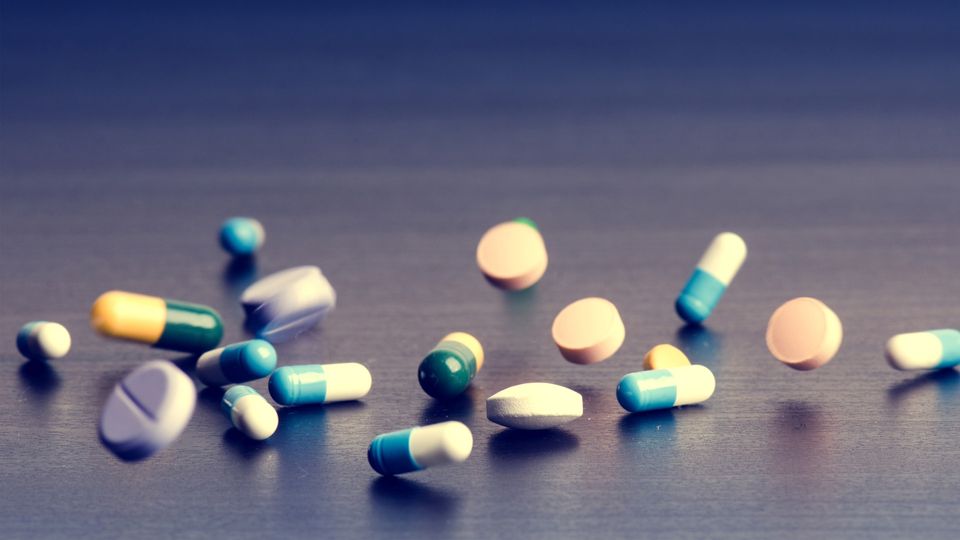
Complete the form below to unlock access to ALL audio articles.
Traditionally, drug discovery programs are carried out by optimizing drug–target affinity and selectivity. Though tremendous progress has been made in this field, attrition rates in drug discovery programs remain high. Around 90% of drug candidates fail during clinical development and almost 60% of all research and development costs are due to attrition.
Lack of in vivo efficacy and toxicity of the drug candidate are common problems encountered during the drug development cycle, as some candidates may show good results in the early stages but fail to show effects in clinical trials. One reason for this failure is that the efficacy of a drug candidate not only depends on the drug–target binding affinity, but also on the drug’s binding kinetics, i.e., the time in which the drug and its target associate and dissociate.
This article will outline why studying drug binding kinetics is important in drug discovery, covering key approaches to measure the drug–target kinetics.
What is drug binding kinetics, and why is it important in drug discovery?
Drug binding kinetics describes how a drug will interact with its target under physiological conditions to produce a desired therapeutic response. It is described by the association rate constant (kon) – the rate at which the drug binds with its target – and the dissociation rate constant (koff) – the rate at which the drug dissociates from the target.
Binding kinetics can influence a drug's efficacy, safety, duration of action and clinical differentiation. Drugs with longer duration of action (that can be administered as a once-daily dose) have slower dissociation constants.
For example, tiotropium – the long-acting bronchodilator – is a non-selective muscarinic antagonist. It can bind to muscarinic receptors M1, M2 and M3, but it dissociates 10 times slower from the M3 receptor. Therefore, it is more selective towards the M3 receptor than M1 and M2 receptors. The selectivity for M3 receptors renders tiotropium its unique clinical advantages. Drugs such as tiotropium work well because of kinetic differences – however, they were not designed that way several years ago. Thus, studying drug binding kinetics earlier in the drug discovery and development programs can help develop drugs with more defined properties.
Furthermore, some studies suggest that the residence time – the total time the drug spends in contact with its target – can help estimate the drug’s in vivo efficacy more effectively than traditionally used thermodynamics parameters such as enthalpy and entropy.
“The human body is an open thermodynamic system and the concentrations of both drug and target can vary as a function of time,” explains Peter Tonge, professor of chemistry at Stony Brook University and director of the Center for Advanced Study of Drug Action. “Consequently, time-dependent target occupancy is a function of both the drug and target concentrations as well as the thermodynamic and kinetic parameters that describe the binding reaction coordinate. Reliance on only thermodynamic parameters such as IC50 values to select and optimize drug candidates fails to take advantage of kinetic selectivity that could contribute to drug efficacy and safety.”
Adriaan IJzerman, professor of medicinal chemistry and head of the medicinal chemistry group at the Division of Drug Discovery & Safety, Leiden University, explains this concept further: “More than a century ago, Paul Ehrlich stipulated that drugs can only act when they are bound to their target in the body. Somehow, thereafter, a general misconception in the world of drug discovery emerged assuming that high affinity for a drug target echoes a long stay of a drug at that target.”
“Measuring the actual kinetics of the interaction proved this wrong: as affinity is the ratio of dissociation and association rate constants, any combination of the two kinetic parameters can yield the same affinity,” IJzerman continues. “Nowadays, we have come to realize that many recently introduced drugs are in fact compounds with a so-called long residence time – they interact with the target for hours or days, ensuring a high target engagement. Hence, it makes sense to study kinetics early in the drug discovery process and incorporate it in the design-make-test-analyze cycle.”
Methods for measuring drug–target binding kinetics
Understanding the atomic interactions between a drug and its target can help identify chemical modifications that can enhance a drug’s kinetic and thermodynamic parameters. Approaches to studying the drug–target binding kinetics are divided into three categories: assays that utilize a label for detection, label-free techniques and assays based on enzyme activity.
Radiolabeling and spectroscopic labeling are the main types of label detection assays. Radiolabeling techniques include techniques such as radiometric ligand binding assays, which can include both direct radiolabeling of the ligand and indirect ways using competitive assays. These are the method of choice for targets such as G protein-coupled receptors.
Additionally, spectroscopic detection methods are usually based on measuring fluorescence, with Förster resonance energy transfer (FRET) and time-resolved FRET (TR-FRET) being two popular approaches for measuring binding kinetics. Both are based on the energy transfer between two different fluorophores that are fused to two interacting proteins. “One relatively recent advance has been the use of methods to quantify binding in live cells,” says Tonge. “Much like [how] green fluorescent protein revolutionized cell and molecular biology, live cell target engagement assays enable binding to be evaluated in the complex milieu of the cell.”
The most popular label-free technique is surface plasmon resonance (SPR), a highly sensitive method to detect biomolecular interactions. SPR detects refractive index changes that occur in response to biomolecular interactions and is especially useful for studying globular proteins. Aside from SPR, other label-free alternatives include the surface acoustic wave method and isothermal titration calorimetry.
Lastly, assays based on enzyme activity are designed to extract kinetic information in a similar way to assays that determine the activity of a specific enzyme. Such enzymatic assays work by measuring the amount of substrate consumption or product formation using spectroscopy methods.
Studying kinetics early can benefit drug development
Understanding and optimizing the binding kinetics can help to design drugs with better therapeutic indexes, thereby decreasing drug attrition. Additionally, numerous companies have started including residence time optimization as a new step in their drug developmental pipelines and this can have a positive impact on highly costly and time-consuming steps.
“These techniques require enhanced sampling at various time points and are often not exactly high throughput, which may be the reason why a kinetic analysis of drug effects is not always part of the discovery process,” IJzerman explains. “Studying kinetics in the early phases, however, may help tremendously in the selection of chemotypes in the hit-to-lead phase and, later, in the tuning of drug candidates during the lead optimization process.”
About the interviewees:
Peter Tonge is a distinguished professor of chemistry and of radiology (by courtesy) at Stony Brook University, and director of the Center for Advanced Study of Drug Action. A primary focus of his program involves the integration of drug-target binding kinetics into predictions of drug activity to improve the selection and optimization of drug candidates.
Ad IJzerman is an emeritus professor of medicinal chemistry at Leiden University, The Netherlands. His main focus over the years has been the large superfamily of G protein-coupled receptors (GPCRs). In 2008 his team co-authored one of the first publications describing the 3D structure of the adenosine A2A receptor, followed by a number of similar high-profile papers.