How To Run a Successful CRISPR Experiment
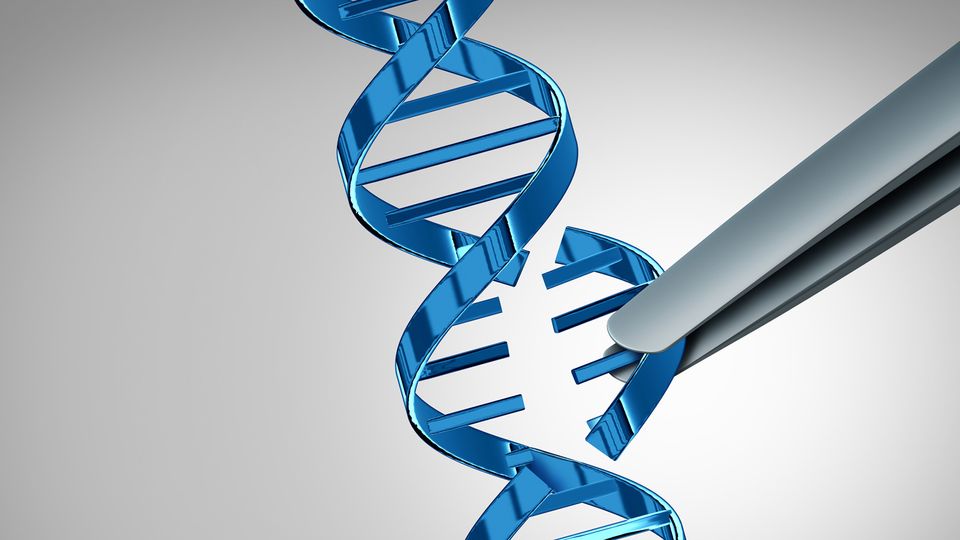
Credit: iStock
CRISPR is a powerful technique that can enhance your research. If you are getting to grips with a CRISPR protocol for the first time, optimizing and planning your experiment can make things easier and can be the key to a successful CRISPR experiment.
Download this guide to learn more about:
- Optimizing CRISPR delivery in your chosen cell line
- Designing your experiment
- Enriching your population
How to Guide
1
How To Run a Successful CRISPR
Experiment
Samantha Carrera, PhD
CRISPR is a powerful technique that can enhance your research. If you are getting to grips with a CRISPR
protocol for the first time, optimizing and planning your experiment can make things easier and can be
the key to a successful CRISPR experiment.
Optimize CRISPR delivery in your chosen cell line
With every new cell line that we use in the lab, we first do an optimization experiment. We trial a few
different transfection conditions (lipofection, nucleofection, etc.) delivering Cas9 and a control guide RNA
that we know consistently work in different cell types. We routinely use a guide targeting the AAVS1 locus
for human cells and tubulin beta for mouse cells. Then, we quantify the editing efficiency using PCR,
Sanger sequencing and inference of CRISPR edits (ICE) analysis and determine the best possible delivery
method for the cell type. Surprisingly, there is a lot of variation in CRISPR efficiency between delivery
methods and cell lines.
You might think that this is too time-consuming, but ultimately you will be saving time and money. At
the end of a CRISPR optimization experiment, you will have a protocol specific for your cell line that you
can use to edit different regions of the genome. Most labs work only with a handful of cell lines, usually
derived from similar tissues, making this step a valuable resource for future work.
Design your experiment - CRISPR knockout
Once you have established the best CRISPR delivery for your cell line of interest, you can select your
desired modification. CRISPR knockouts are the easiest experiments, and they require two components:
Cas9 protein and guide RNA.
When I first started using CRISPR cell lines, we used to deliver Cas9 and guide RNAs as plasmids. Now,
we know that the most efficient method is to use Cas9 proteins and chemically synthesized guide RNAs,
which are preassembled into a ribonucleoprotein (RNP) and delivered into the cells.1 Even though they
are more expensive to buy, it will be more cost-effective in the end as you will save time and effort by not
having to repeat your experiment.
The most important part to design is the guide RNA; there are numerous websites where you can input
your sequence of interest, and they will give you a list of guide RNAs that you can use. The tricky part is
deciding which one to choose.
HOW TO RUN A SUCCESSFUL CRISPR EXPERIMENT 2
How to Guide
First, you must decide which exon of your gene you want to target. I recommend avoiding the first exon if
possible. When you create insertions/deletions (indels) here, the splicing machinery can skip the exon and
still produce a truncated version of the protein.2 We usually pick exons that are as upstream as possible
and that are common to all isoforms. The most common modification introduced by Cas9 is +/- 1 bp, so
directing a guide to exons with an asymmetric splicing phase makes it more likely for the transcript to be
knocked out of frame.
With every CRISPR design, you should pick guides that have low off-targeting potential. Even though
some guides are labelled as inefficient, it is difficult to predict the efficiency of the guide RNA. For this
reason, I would recommend designing three guides and delivering them in parallel alongside the control
guide RNAs (either AAVS1 or tubulin).
Once you have transfected cells for a gene knockout, you can isolate genomic DNA and use primers
designed around the cutting area to determine the percentage of indels you have in your cell population
using Sanger sequencing and ICE/TIDE analysis. Once you have determined this, you will have to do some
functional validations to determine whether your protein of interest has been knocked down; this can be
done by western blotting.
Design your experiment - CRISPR knock-in
I believe that CRISPR knock-ins are where you really realize how powerful this technique is. You can add
functional tags to your gene of interest, including fluorescent proteins, luminescent reporters, proximity
labelling enzymes, degron tags and pull-down tags. You can also insert transgenes in safe harbor loci, socalled
because they aren’t easily silenced. These transgenes are used in technology platforms that allow
you to control the expression of your protein of interest by adding small molecules. These include degron
systems and tetOn/Off compatible cells. You can also introduce point mutations into your gene of interest
or into enhancer sequences to study gene regulation.
A CRISPR knock-in experiment is harder to plan than a knockout experiment, and decisions need to be
made about the site of insertion and the need for additional tags and linker sequences. If you are introducing
functional tags, you might need to first assess if N- or C- terminal tagging is better for preserving
the function and expression of your protein. Once you have decided this, you must design a guide that is
close to the site (within 20 bp) where you want your insertion.
The next step is to design your homology directed repair (HDR) template, which will contain your insert of
interest flanked by homology arms. The homology arms are sequences of DNA that correspond to your
gene of interest on either side of your insertion site. It is worth remembering that the larger the insert,
the more inefficient the HDR process is going to be and the longer homology arms you will need.
If your insertion is small (<100 bp), the best thing to use is a single-stranded DNA template with ~80 bplong
homology arms on each side. These are commercially available and usually have modifications that
make them more likely to get integrated in the genome. For larger insertions we prefer using plasmids
where we clone the homology arms and our insert. The homology arms in this case are around 800 bp.
It is important to make sure to mutate the protospacer adjacent motif (PAM) site in the HDR template to
avoid Cas9 cutting it (causing insertions or deletions).
Once you have designed your guides and your template, you can use your optimized protocol to deliver
them into your cells and create a polyclonal population. At this point, you will have many cells that have
not been modified and it is worth spending some time considering how to enrich your pool of cells with
your desired modification.
HOW TO RUN A SUCCESSFUL CRISPR EXPERIMENT 3
How to Guide
Enrich your modification
The next thing that you need to consider is if a polyclonal cell line is sufficient for your experiments or if
you need to go through the labour-intensive process of generating a monoclonal cell line.
If your desired edit contains a sortable marker, like a fluorophore, you can first enrich your population
of cells by fluorescence-activated cell sorting (FACS). This will result in a pool of cells that are slightly
different from each other but which all express your fluorophore. It is good to bear in mind that the levels
of fluorescence will depend on the expression of your gene of interest, so it might be hard to distinguish a
positive population if the levels are too low.
Another way of enriching your population is by introducing an antibiotic selection cassette and treating
the cells with that antibiotic. This will result in the elimination of the cells without the insert, leaving behind
a positive pool of cells.
Sometimes you don’t want your protein to have a fluorophore or an antibiotic cassette that could potentially
interfere with its function. In this case, we co-integrate a removable antibiotic selection cassette
which allows us to select for HDR-positive cells. A promoter and the desired antibiotic are flanked by loxP
sites that allow their removal once the cells have been verified by expressing Cre-recombinase (Figure 1).
With the fluorophores you can introduce a cleavable signal like T2A, in that way your protein of interest
and the fluorophore will be cleaved when transduced, making it less likely that the insertion interferes
with the function of the protein of interest.
Gene of interest
gRNA_1
5'H 3'H
gRNA_2
Cas9 HDR template
Gene of interest
Gene of interest
5'H with PAM mutations mScarlet-I 3'H
5'H with PAM mutations mScarlet-I
PGK promoter bGH poly(A) signal
PuroR 3'H
Cre recombinase
IoxP IoxP
T2A
7500
IoxP
7500 10,000
2000 3000
Figure 1: CRISPR knock-in enrichment by removable antibiotic selection. Cas9 is delivered into the cells along with
two guide RNAs (gRNA) and an HDR template. The HDR template includes a Puromycin resistance cassette that
will allow the selection of cells that had integrated the fluorophore (mScarlet-I). Once the cells have been selected,
the antibiotic selection cassette can be removed by the addition of Cre recombinase. Credit: Technology Networks
HOW TO RUN A SUCCESSFUL CRISPR EXPERIMENT 4
How to Guide
If you want to produce monoclonal cell lines, you can use your enriched population and either FACS sort
into single cells or use the serial dilution method. This method consists in diluting your cells until you
have 1 cell for each 100 μl and seed this volume into a single well on a 96-well plate. It is important to
note that not all cell lines are happy growing as single cells and you will struggle to create monoclonal
populations out of these cell lines. In my experience with these cells, it is worth seeding the single cells
into conditional media – this is media taken from a growing population of cells that has been filtered to
avoid carrying any cells but with the advantage of carrying any secreted proteins.
Finally, you need to verify that your cells have the desired modification. For this, there are a few things
you must consider. First, designing your primers outside of the homology arms to discard the possibility
that your template has integrated in another area of the genome. You also must remember that your PCR
will preferentially amplify any unmodified alleles, as it requires less time to copy a smaller DNA product
than a bigger one. I usually design a handful of primers including internal ones and insert-specific ones
that I can use in different combinations. This will give a good indication that the cells have the desired
insert in them. I will always send these PCR products for sequencing to check that there are no insertions,
deletions or mutations that will interfere with the expression of my gene. You will also need to do some
functional validations, for example using western blotting to see if your protein has a size shift that corresponds
to your insert.
Conclusion
Creating CRISPR cell lines doesn’t have to be a difficult process. If you take advantage of the tips above,
you can create all the tools you need to advance your research, opening different possibilities for studying
your prefered cellular pathway. With all these things in mind, enjoy CRISPRing!
About the Author:
Samantha Carrera is originally from Mexico and completed her PhD in Biochemistry at the University of Leicester in the UK where she
studied the effect of the microenvironment on the response to apoptosis. After graduating, she worked as a postdoctoral scientist at
Bristol University researching transcription factor regulation. For her second postdoc, she moved to Manchester University to study
gene regulation. In 2020, she started work as an experimental officer in the cell engineering branch of the Genome Editing Unit Core
Facility at the University of Manchester where she utilises CRISPR and other technologies to create cell lines for research groups
across the university.
Sponsored by
References
1. Kim S, Kim D, Cho SW, Kim J, Kim JS. Highly efficient RNA-guided genome editing in human cells via delivery of purified
Cas9 ribonucleoproteins. Genome Res. 2014;24(6):1012-1019. doi: 10.1101/gr.171322.113
2. Sharpe JJ, Cooper TA. Unexpected consequences: exon skipping caused by CRISPR-generated mutations. Genome Biol.
2017;18(1):109. doi:10.1186/s13059-017-1240-0
Sponsored by
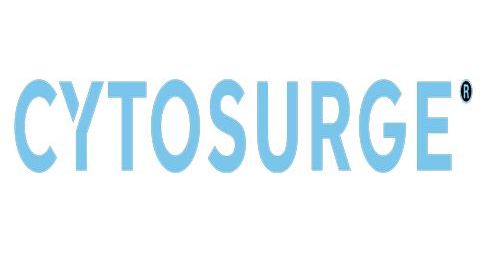
Download the How To Guide for FREE Now!
Information you provide will be shared with the sponsors for this content. Technology Networks or its sponsors may contact you to offer you content or products based on your interest in this topic. You may opt-out at any time.