Benchtop NMR Breaks New Ground
NMR can now be used in a wider range of applications, by non-expert users in standard laboratory settings, and even in process and reaction monitoring and control scenarios.
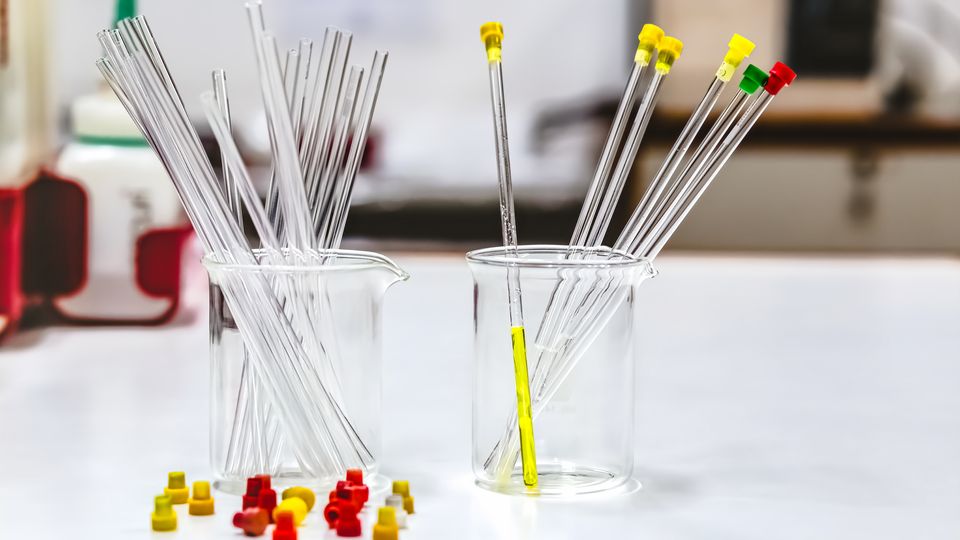
Complete the form below to unlock access to ALL audio articles.
Nuclear magnetic resonance (NMR) spectroscopy is recognized as an advanced analytical technique that provides unique information for chemists, biochemists and materials scientists. It is a powerful tool for the elucidation of molecular structure in solution, and is used for the identification, structural characterization and quantification of compounds.
NMR provides valuable insight into the chemical environment, bonding and connectivity within a molecule. It is a non-destructive technique, is inherently quantitative and does not require dedicated reference/calibration samples.1 NMR is highly prized in fields such as organic chemistry, synthetic chemistry and biopharmaceutical development, which require detailed analysis of molecular structure.
NMR spectroscopy is best known in the context of so-called high-field NMR – in which the instruments use large superconducting magnets to generate high field strength for maximum sensitivity and resolution of the measurement. These systems, which are mostly located in academic research facilities or industrial analytical labs, require dedicated infrastructure and services (liquid helium and nitrogen), and expert users. They are often too expensive or too complex – or both – for a routine analytical lab to purchase, run and maintain. For those scientists who have access to a dedicated facility, the time taken to get their samples analyzed can be a key barrier.
However, many areas of routine analysis would benefit from NMR, with some experts even suggesting that NMR could eventually become a tool for point-of-care diagnostics in metabolic conditions.2 The full potential of the technique is only likely to be realized if the issues of instrument accessibility, speed of analysis, sample work-up and ease of use are addressed.
Advantages of benchtop NMR
Compared to a high-field NMR spectrometer, the most obvious difference with a benchtop system is, of course, its size. It is important to note, however, that to reduce instrument size, system designers and engineers had to overcome a series of challenges and rethink several of the fundamentals of making an NMR measurement. Early attempts to introduce benchtop systems failed to deliver the required performance but more recently, over the last decade or so, a series of developments have allowed a new generation of benchtop NMR instruments to successfully come to market – to positive reviews. Not all benchtop NMR systems on the market today are equal, but for those that have overcome the most difficulties, their use is becoming more and more routine. Now, having the NMR spectrometers in chemistry labs substantially shortened the time required to analyze the samples. This opened up the possibility of using these instruments for on-line analysis. The main challenges for these applications are due to the protonated solvents typically used in the reactors.
NMR spectroscopy requires the samples to be in solution to allow measurement, and the solvents commonly used in the process of synthesizing the compound to be tested most often have NMR resonances that are orders of magnitude larger than the signal of the sample being measured. To overcome this, the current standard procedure requires the original protonated solvent to be replaced with a deuterated alternative, which eliminates the dominant solvent signal. As a result, a demanding and time-consuming sample work-up process that requires solvent evaporation and redissolution must be performed before each sample can be measured. This basic requirement is not conducive to easy access to NMR instruments, nor speedy analysis.
Challenge 1: efficient solvent suppression
Highly selective solvent suppression methods, like PRESAT or WET, are very successful in high field magnets, but have remained a challenge for benchtop systems because high magnetic field homogeneity is required to achieve an efficient solvent suppression. However, recent years have seen the development of permanent magnets3 that operate at 60, 80 or 90 MHz – and offer superior homogeneity – which has helped to overcome this challenge. In these ultra-high homogeneous magnetic fields, the signals of the solvents can be selectively suppressed to remove the overlapping of these large peaks with the signals of the products.
Challenge 2: an alternative “lock”
Besides eliminating the solvent signal, deuterated solvents have a second and very important function in high-field NMR spectrometers: the deuterium signal is used to track and correct the drift of the magnetic field generated by the magnet. By “locking” the magnetic field in this way, the high field stability required for the measurement is achieved.
Now, modern benchtop NMR instruments utilize a “lock system” which relies on a capillary sample mounted permanently in the magnet – independent of the sample under test. This means that the need for deuterated solvents to lock the magnetic field is eliminated and samples can be analyzed (in protonated solvents) without sample preparation. This move enables sample-to-test timing to be achieved, which would be unthinkable with high-field instruments.
To summarize: by removing the need for deuterated samples, and successfully applying solvent suppression methodology at low magnetic fields, the full power of benchtop NMR has been leveraged. Analysis is rapid, the footprint of instruments can be dramatically reduced, and the cost and burden of running a high-field system is removed.
One application where chemists have rapidly adopted the new-generation instruments is reaction monitoring and control.
Whether a user is manually sampling at various points of a process, or is pumping directly from a reactor into the spectrometer, benchtop NMR can now be used to monitor:
• batch reactors to understand the kinetics of the reaction, allow better management of the reaction, and decide when the reaction has been completed.
• continuous flow reactors for measuring product quality in real-time.
In practice
A recently published example of how NMR is being used in an automated closed-loop system with batch reactors is shown below.
The closed-loop approach automates chemical exploration through trial and error using a decision-making algorithm and online analytics. It requires three components: a chemical robot to carry out analysis, a program for data interpretation, and an algorithm that links reaction outcomes to the input and process parameters and suggests appropriate parameters for the next reactions (see Figure 1).
To enable autonomous reactivity-first discovery, both the analytical approach and reactivity detection algorithm must be general-purpose and robust; the speed and small size of benchtop NMR systems have facilitated this in situ monitoring.
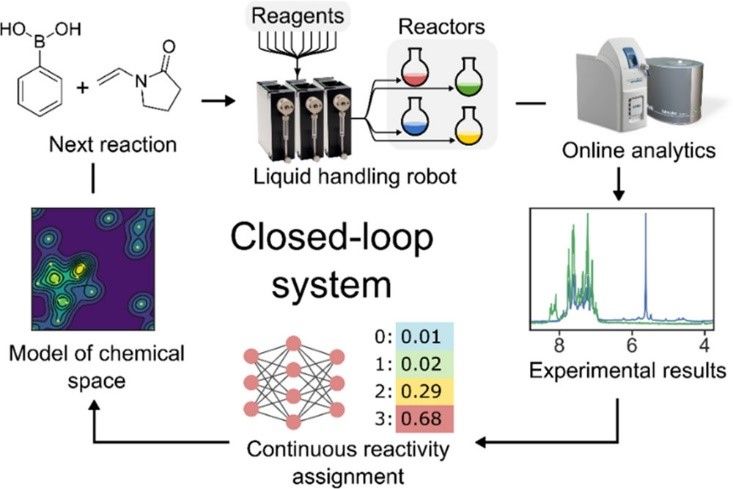
Figure 1: Closed-loop framework for chemical space exploration. A liquid handling robot performs an experiment and collects NMR and MS spectra. These data are processed to assess reactivity and create a model of the chemical space that is queried to formulate the next experiment to be performed.4 Credit: Caramelli D, Granda JM, Mehr SHM, Cambié D, Henson AB, Cronin L. 2021. CC BY 4.0.
Looking ahead
Benchtop NMR is now set to bring the power of NMR to wider application in routine labs – especially those systems that offer superior magnet homogeneity and enhanced solvent suppression.
Moreover, the innovation and technical developments that have enabled the dramatic shift in performance, accessibility and ease of use are establishing NMR in batch and continuous flow reaction monitoring systems, helping to drive forward autonomous discovery.
In future, scientists looking for easier, faster and less expensive structural analysis and compound quantification when working with, for example, polymers, forensic drug analysis, food science and small molecule drug development, will surely find a place on their bench for an NMR spectrometer.
About the author:
Jürgen Kolz is the head of the Application Development team at Magritek GmbH. He received a diploma in chemical engineering from Aachen University of Applied Sciences in 2004 and completed his PhD in chemistry in the group of Professor Bernhard Blümich at RWTH Aachen University in 2008. This was followed by a postdoctoral stay at the University of Cambridge in the group of Lynn Gladden. In 2010, he returned to Aachen and joined Magritek, where he is now responsible for a team of chemists involved in applications development and customer support.
References
1. Emwas AHM. The strengths and weaknesses of NMR spectroscopy and mass spectrometry with particular focus on metabolomics research. In: Bjerrum JT, ed. Metabonomics. Vol 1277. Springer New York; 2015:161-193. doi: 10.1007/978-1-4939-2377-9_13
2. Percival BC, Grootveld M, Gibson M, et al. Low-field, benchtop NMR spectroscopy as a potential tool for point-of-care diagnostics of metabolic conditions: validation, protocols and computational models. High-Throughput. 2018;8(1):2. doi: 10.3390/ht8010002
3. Solvent suppression: the key to measure your samples every time you need while they are synthesized. Magritek. https://magritek.com/2022/03/24/solvent-suppression-the-key-to-measure-your-samples-immediately-while-they-are-synthesized/. Published March 24, 2022. Accessed November 3, 2023.
4. Caramelli D, Granda JM, Mehr SHM, Cambié D, Henson AB, Cronin L. Discovering new chemistry with an autonomous robotic platform driven by a reactivity-seeking neural network. ACS Cent Sci. 2021;7(11):1821-1830. doi: 10.1021/acscentsci.1c00435