The Scientific Observer Issue 32
Magazine
Published: November 24, 2023
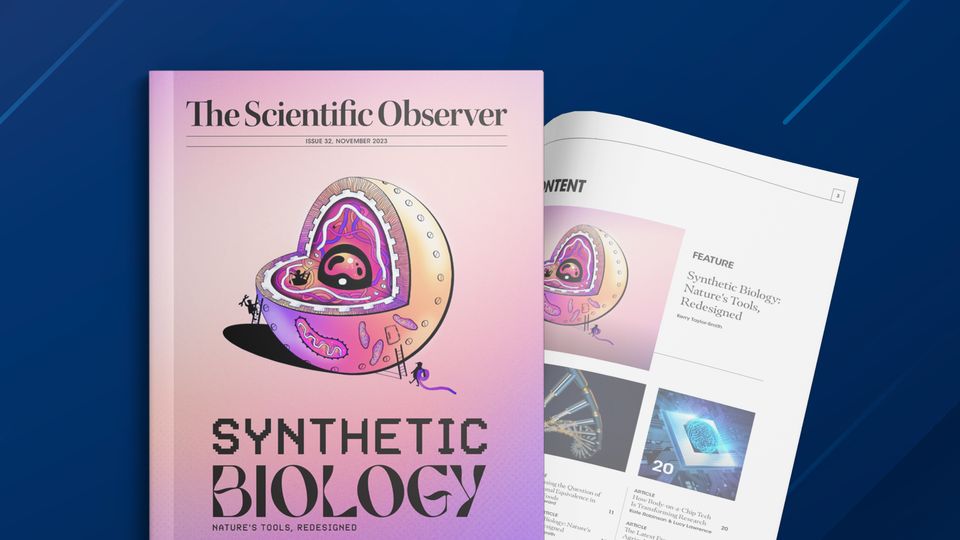
Credit: Technology Networks
In this issue of The Scientific Observer, we’re focusing on synthetic biology – a field that fuses biology and engineering to produce biological systems with novel abilities. Find out how nature’s tools – when redesigned – can support humanity’s efforts to develop therapeutics, combat global issues such as climate change and much more.
Issue 32 highlights:
- Half-Synthetic Yeast Engineered for the First Time
- How Body-on-a-Chip Tech Is Transforming Research
- Synthetic Biology: Nature’s Tools, Redesigned
Half-Synthetic Yeast
Engineered for the First Time
How Body-on-a-Chip Is
Transforming Research
ISSUE 32, NOVEMBER 2023
Biology
NATURE'S TOOLS, REDESIGNED
2
CONTENT
FROM THE NEWSROOM 04
ARTICLE
Half-Synthetic Yeast Engineered
for the First Time 06
Molly Campbell
RESEARCH SPOTLIGHT
Implanting Microdevices Into
Brain Tumors To Determine the
Best Treatment 09
Suhanee Mitragotri
ARTICLE
Addressing the Question of
Nutritional Equivalence in
Future Foods 11
Karen Steward
FEATURE ARTICLE
Synthetic Biology: Nature's
Tools, Redesigned 15
Kerry Taylor-Smith
ARTICLE
How Body-on-a-Chip Tech
Is Transforming Research 20
Kate Robinson & Lucy Lawrence
ARTICLE
The Latest Frontiers in
Agricultural Science 24
Molly Campbell
06 20
15
FEATURE
Synthetic Biology:
Nature's Tools,
Redesigned
Kerry Taylor-Smith
AnnaMaria Vasco, iStock
3
Kate Robinson
Kate Robinson is an
Assistant Editor at
Technology Networks.
EDITORS’ NOTE CONTRIBUTORS
Have an idea for a story?
If you would like to contribute to
The Scientific Observer, please
feel free to email our friendly
editorial team.
Kerry Taylor-Smith
Kerry Taylor-Smith is a
Science Writer who covers
everything from climate
change and the environment to health and wellness,
to material science,
astronomy and space.
Karen Steward, PhD
Karen is a Senior
Science Specialist for
Technology Networks.
Lucy Lawrence
Lucy is a Senior Digital
Content Producer at
Technology Networks.
Molly Campbell
Molly is a Senior
Science Writer at
Technology Networks.
Suhanee Mitragotri
Suhanee is a student
at Harvard University,
majoring in neuroscience
with a minor in global
health and health policy.
Dear Readers,
Welcome to issue 32 of The Scientific Observer, the monthly
magazine brought to you by Technology Networks.
From the Technology Networks newsroom, we bring you
a curation of the most interesting research stories from
the last month. Did you know that female chimpanzees
can experience menopause? Or that your favorite music
might help to sooth the experience of pain? From alarming
pesticide research in Sri Lanka to a world-first virology
discovery at the University of Pittsburgh, this issue’s news
highlights aren’t to be missed.
The intersection of science and agriculture will be critical
for addressing our planet’s growing population and the
effects of climate change. Synthetic biology – a field that
fuses biology and engineering – will undoubtedly play a
profound role, offering innovative solutions to enhance
crop resilience, increase yields and minimize the environmental footprint of agriculture. In this month’s feature
article, Kerry Taylor-Smith explores how nature’s tools
– when redesigned – can support humanity’s efforts to
combat some of our biggest global issues.
Also in issue 32, Molly Campbell reports on a recent
announcement from The Synthetic Yeast Genome Project
2.0. The project – which aims to synthesize an entirely
synthetic yeast genome – is now halfway to achieving its
goal after engineering yeast that has an over half synthetic
genome. The milestone brings us one step closer to harnessing synthetic genomes for a multitude of purposes,
such as the production of medicines or biofuels.
This, and much more, in issue 32 of The Scientific Observer.
The Technology Networks Editorial Team
4
From the Newsroom
FROM THE NEWSROOM
In a first-of-its-kind discovery, scientists visualize a satellite
virus latching onto a helper virus.
JOURNAL: Journal of the International Society of Microbial Ecology
Viruses Can Latch Onto
One Another
MOLLY CAMPBELL
Your favorite music might be good for more than a workout
soundtrack or late-night listening session, suggests a new study.
The research suggests that individuals’ most-loved music could
reduce feelings of pain compared to relaxing stock music.
JOURNAL: Frontiers in Pain Research
Pain-Busting Ballads: Researchers
Identify Music That Best Soothes
Pain
RUAIRI J MACKENZIE
Female chimpanzees in the wild can experience menopause and
survival past their reproductive years in a similar way to humans,
reveals a new study that could help researchers to understand
why menopause and post-fertile survival occur in nature as well
as its evolution in humans.
JOURNAL: Science
Wild Chimpanzees Can Experience
Menopause Similar to Humans
SARAH WHELAN
5 FROM THE NEWSROOM 5
Want to learn more?
Check out the Technology Networks newsroom.
A new study suggests that the active ingredient in Roundup
– the world’s most popular herbicide – may play a role in the epidemic levels of chronic kidney disease seen in rural Sri Lanka.
JOURNAL: Environmental Science and Technology Letters
Popular Herbicide Ingredient
Linked to Chronic Kidney Disease
Epidemic in Sri Lanka
ALEX BEADLE
In a new paper, researchers say they have found “definitive”
archeological evidence that seaweeds and other freshwater
plants were eaten in Europe from the Mesolithic period to the
early Middle Ages.
JOURNAL: Nature Communications
Ancient Europeans Regularly
Ate Seaweed
LEO BEAR-MCGUINNESS
6
SCIENTISTS ENGINEER A STRAIN
OF YEAST THAT HAS AN OVER
HALF SYNTHETIC GENOME.
T
he Synthetic Yeast Genome
Project 2.0 (Sc2.0) is a
global project working to
synthesize the entire yeast
genome. In a collection of papers
spanning Cell, Molecular Cell and
Cell Genomics, the project declares
a major milestone: it has created a
yeast strain comprising over 50%
synthetic DNA.
GENERATING SYNTHETIC
GENOMES
Synthetic genomes are created by
designing and assembling DNA
sequences that are then inserted
into an organism’s cells, effectively
allowing researchers to “customize”
the organism with certain characteristics. It’s an area of research
within synthetic biology that utilizes recent advances in molecular
biology, genetic engineering and
gene synthesis.
“Writing a genome is in many
ways a test of how well you understand its function and its
evolved natural ‘design’, says
Dr. Jef Boeke, a synthetic biologist
at New York University (NYU)
Langone Health and the leader
of Sc2.0.
The first efforts to generate synthetic
genomes largely focused on viruses
or bacteria. In 2010, scientists
from the J. Craig Venter Institute
synthesized the first synthetic bacHalf-Synthetic Yeast Engineered for
the First Time
MOLLY CAMPBELL
iStock
terial genome Mycoplasma mycoides
JCVI-syn1.0.
Boeke and colleagues have been
working hard on Sc2.0 for at least
18 years, as synthesizing a yeast
genome is more technologically
challenging than a bacterial genome.
“It’s substantially bigger. It’s more
repetitive than bacterial genomes,
and it’s made up of many individual
chromosomes, whereas most bacteria have only one,” Boeke explains.
It’s a challenge that is worthwhile
in Boeke’s mind, considering that
humans are more closely related to
yeast than bacteria, making yeast a
better model for understanding how
human cells work.
YEAST’S DESIGNER GENOME
The half-synthetic yeast has a “designer” genome that is based on Saccharomyces cerevisiae (S. cerevisiae),
commonly referred to as baker’s or
brewer’s yeast. Boeke and colleagues
wanted to create a strain that could
help them understand how the model
organism functions and how it could
be improved. It was therefore important that the synthetic yeast was
highly modified.
Non-coding and repetitive elements
of DNA were removed and a diversity
generator called Synthetic Chromosome Recombination and Modification by LoxP-mediated Evolution, or
the “SCRaMbLE” system, was added.
Boeke and colleagues also created a
new chromosome, which does not exist in nature. Genes encoding transfer R NA (tR NA) are often associated
with regulatory elements that can
lead to stability issues when integrated into a synthetic chromosome.
The researchers decided to remove
these genes and created a tRNA “neochromosome”.
“The tR NA neochromosome is not
modeled on an existing natural chromosome but was literally designed
piece by piece, on a computer, and
the pieces came from at least 5 different species of microorganisms,” says
Boeke. “The fact that it can function,
by producing tR NAs like a natural
genome does, is pretty amazing. It is
also designed to avoid ‘head-on collisions’ between R NA polymerase
and DNA polymerase which can lead
to DNA breaks and other challenges
for the cell.”
STRAIN GENERATED THAT IS
OVER 50% SYNTHETIC
S. cerevisiae’s genome is organized
into 16 chromosomes, with each
team in the consortium responsible
for producing one synthetic chromosome at a time. “Sc2.0 started small
and grew to be a project involving researchers in four continents and nine
countries,” says Boeke. The teams
independently assembled each chromosome to produce 16 strains, each
of which comprised 15 native chromosomes and one synthetic chromosome. The next challenge was finding
a way to pull all of the synthetic
chromosomes together.
The researchers developed a new
method called chromosome substitution to bring the synthetic chromosomes together in a single strain. “We
combined two methods to do this:
transfer of the synthetic chromosome
to a recipient strain by doing a special
kind of genetic cross. In this cross, a
single chromosome moves from one
nucleus to the other – a process called
‘chromoduction’,” explains Boeke.
“This leads to a cell with one extra
synthetic chromosome. At this point,
the resident natural chromosome
corresponding to it can be destabilized by forcing R NA polymerase to
traverse its centromere, a process
that greatly destabilizes it. Et voila!
A cell with a synthetic chromosome
swapped in place of a native chromosome.” By integrating seven of the
synthetic chromosomes, Sc2.0 has
succeeded in generating a strain that
is over 50% synthetic.
The synthetic yeast strain was found
to possess genetic defects, or “bugs”,
such as genetic interactions between
genes located on the synthetic chromosomes. Such issues had been anticipated, so Boeke and colleagues were
prepared to map the bugs and find a
fix. “The defects can result from R NA
Scanning electron micrographs of the syn6.5 strain of yeast which has ~31% synthetic DNA and displays
normal morphology and budding behavior.
7
Cell/Zhao et al
8
iStock
not folding up as it should,” says
Boeke. “Once mapped, bugs can be
easily corrected by removing the
changed bases and restoring the native sequence. This has already been
done for every bug.”
A MAJOR MILESTONE
The collection of papers marks a
milestone in genomics and synthetic
biology, but the real fun will begin
once the rest of the synthetic chromosomes are integrated. “That’s when
we’re really going to be able to start
shuffling that deck and producing
yeast that can do things that we’ve
never seen before,” says Boeke.
Synthetic genomes designed for the
production of medicines or biofuels
might well become a reality in the near
future – the foundations are being
laid, after all.
Boeke is often asked, “which genome
is next”? His team at NYU Langone
Health is currently working on “The
Dark Matter Project”, which seeks
to understand what new information
lies in the non-protein-coding portion
of the mammalian genome. ⚫
Dr. Jef Boeke was speaking to Molly
Campbell, Senior Science Writer for
Technology Networks.
References
1. Zhao Y, Coelho C, Hughes A, et al. Debugging and consolidating multiple synthetic
chromosomes reveals combinatorial genetic
interactions. Cell. 2023. doi: 10.1016/j.
cell.2023.09.025
2. Schindler D, Walker R, Jiang S, et al., Design,
construction, and functional characterization of a tR NA neochromosome in yeast.
Cell. 2023. doi: 10.1016/j.cell.2023.10.015
3. Shen Y, Gao F, Wang Y, et al. Dissecting aneuploidy phenotypes by constructing Sc2.0
chromosome VII and SCRaMbLEing synthetic disomic yeast. Cell Genomics. 2023.
doi: 10.1016/j.xgen.2023.100364
4. McCulloch L, Sambasivam V, Hughes A, et al.
Consequences of a telomerase-related fitness
defect and chromosome substitution technology in yeast synIX strains. Cell Genomics.
2023. doi: 10.1016/j.xgen.2023.100419
WANT TO LEARN MORE ABOUT SYNTHETIC BIOLOGY?
Visit the Technology Networks synthetic biology topic hub, where you
can access educational resources, events, news and products related
to synthetic biology.
9
iStock
Implanting Microdevices
Into Brain Tumors To
Determine the Best
Treatment
Suhanee Mitragotri
RESEARCH SPOTLIGHT
Malignant brain tumors account for one to two percent of all types of
cancers in adults and remain one of the hardest types of cancer to treat. A
multi-institutional study published in Science Translational Medicine,
led by Dr. Pierpaolo Peruzzi at Brigham and Women’s Hospital,
demonstrates the potential for using intratumoral microdevices
(IMDs) to test various cancer drugs on the tumor before implementing
systemic treatment.
iStock
9
Research
Spotlight
10
iStock
THE CHALLENGE OF
HETEROGENEITY IN BRAIN
TUMORS
Gliomas, one of the most aggressive
forms of brain tumors in humans,
have been researched heavily over
the past decades and various genes
have been implicated in this cancer,
including EGFR, PDGFRA, and TP53.
Therapies have been developed to
target these genes and inhibit cancer
growth and proliferation. While
these therapies have been successful
in preclinical models, many of them
have shown variable success in clinical testing, largely because every
glioma has a distinct genetic makeup
and developing therapies that can
target mutant genes in all patients is
extremely difficult. Therefore, it has
become evident that cancer treatments for patients would be most
effective if they are created based
on the specific genetic profile of the
tumor at hand. Inspired by this era of
personalized medicine, Peruzzi et al.
developed tiny biocompatible IMDs
that can be inserted into the tumor
at the time of surgery and release
small doses of different drugs at
distinct points in the surrounding
tissue over the course of two hours.
Afterwards, the tissue can be analyzed to measure the impact of
each individual drug on the cancer’s
growth and proliferation.
ASSESSING SAFETY
AND EFFICACY OF
MICRODEVICES IN
DELIVERING DRUGS
Six patients diagnosed with brain
tumors were enrolled in the study
(50% female, 50% male). The median age of the participants was 76
years, with a range between 27 and
86 years of age. All patients were
already scheduled to undergo tumor
resection surgery during which each
had two IMDs implanted at the tumor site, giving a total of implanted
12 IMDs. The researchers delivered
nine drugs through the IMDs, specifically temozolomide, doxorubicin,
lapatinib, lomustine, irinotecan,
carboplatin, osimertinib, abemaciclib
and everolimus. They measured the
safety of the IMDs in all patients and
analyzed the feasibility of conducting
molecular analysis on the tissue
after surgery. They assessed DNA
damage (via pH2AX expression) and
apoptosis (via CC3 expression) in
the tissue surrounding the IMD, as
well as measuring how distance from
the implantation site impacted the
degree of drug effect.
The key findings of the paper
were:
• The IMD implantation resulted in no adverse events or
significant changes in surgical
outcomes or cost, and 11 out of
the 12 implantations resulted in
tissue specimens that could be
successfully processed in molecular analysis.
• The greatest degree of DNA
damage in the surrounding tissue was found in patient 3, where
65.8% of the cells were impacted, and the lowest amount was
found in patient 5, where 9.8% of
the cells were impacted.
• Apoptosis induction was generally low (< 5%), except in patient
3, where it was measured in
13.1% of the cells.
• The greatest DNA damage was
found in tissue near the IMD site
and was found to decrease with
lower doses.
• Changes in gene transcription
on a drug-to-drug basis correlated with the pathways each drug
targeted, confirming that the
drugs worked as expected.
ANALYZING TISSUE FROM
THE IMD SITE TO DETERMINE
DRUG EFFICACY
Dr. Peruzzi and his team have
demonstrated the potential that
IMDs have in quickly assessing the
best treatment for a specific patient’s
brain tumor. The implementation of
IMDs was safe in all patients and
did not impact the outcomes of surgical procedures or increase surgical
costs significantly. By measuring the
amount of DNA damage and apoptosis in the tissue surrounding the
IMD, the researchers were able to
assess the impact that each drug had
on cancer progression, as each drug
was released in a distinct location
within the tissue. A variable range of
DNA damage was seen from patient
to patient, which could be due to the
heterogeneity of tumors. Furthermore, DNA damage appeared to be
concentrated at the IMD site, indicating that the delivery of treatment
was localized.
While this study displays the promise that IMDs could have in the treatment of brain tumors, there are limitations to the work. Firstly, since the
IMD implantation was conducted in
patients who were already undergoing scheduled surgery, the IMD could
only be inside the tumor for around
two hours, which may not have been
enough time to observe changes in
markers for apoptosis. Furthermore,
the study consisted of a small population size, and larger clinical studies
will need to be conducted to confirm
the predictive capability of IMDs.
THE NEED FOR LARGER AND
LONGER CLINICAL STUDIES
Brain tumors remain one of the
hardest cancers to treat, largely due
to their heterogeneity, but Peruzzi et
al. have developed a viable technology that can provide physicians with
information as to what treatments
are the most effective for a given patient’s tumor. Larger clinical studies
that build upon this research should
consider ways to leave the IMD in
the tumor for longer periods of time,
to maximize the effect that these
drugs can have on the surrounding
tissue and provide doctors with more
accurate information regarding their
efficacy. In an era of personalized
medicine, IMDs could hold the key
for the future of cancer treatment
and clinical care. ⚫
RESEARCH SPOTLIGHT 10
11
iStock
ALTERNATIVE PROTEINS CAN BE
A SUSTAINABLE SUBSTITUTE FOR
MEAT BUT CONSUMERS SHOULD
BE AWARE OF NUTRITIONAL
VARIABILITY
T
here is no denying that our
food supply chain is a system under stress with our
ever-expanding population,
compounded by a changing climate
that’s squeezing producers to maximize production under increasingly
challenging circumstances. And then
there’s the environmental impact
that agriculture itself is having,
with livestock rearing fingered as a
significant contributor, feeding back
into a cyclical problem. It is therefore
no wonder that the research into
and appetite for alternative protein
sources has exploded in the last few
years. Multiple avenues are being
explored, some more novel and ambitious than others, including microbial
fermentation, cellular agriculture,
mycoproteins, insects, algae and
plant-based proteins.
While the likes of cellular agriculture
are still providing the consumer with
animal cells and the nutritional value
they convey, the same cannot necessarily be said for their plant-based
counterparts.
THE LABELING ILLUSION
Look at most food packaging and it
will provide you with a breakdown of
the nutritional values of that product.
However, how many of us really understand what we are being shown or
what’s being hidden? While nutritional breakdowns may give us an idea of
the number of grams of saturated fat
something contains or its calorific
value, we are only seeing part of the
picture. Labels generally state the
protein content grouped together as
a single entity, but proteins are made
up of amino acids, the composition of
which can vary greatly from protein
to protein. This fact is vitally important when we consider our dietary
needs. While our bodies are able to
synthesize some amino acids, others
must be ingested in the food we eat,
hence it is important that we include
protein sources rich in these missing
“essential amino acids” in our diet.
But looking at most packaging, it is
Addressing the Question of
Nutritional Equivalence in Future
Foods
KAREN STEWARD
12
iStock
not possible to tell whether the amino acids obtained from a plant-based
burger, for example, are equivalent to
those we could obtain from eating its
meat-based counterpart.
The amino acid make-up is not the
only aspect that may vary either.
Digestibility, a factor impacted by
how the building blocks of molecules
are put together in those foods, can
have a great impact on what we can or
cannot obtain from it. The presence
of so called “antinutrients”, such as
phytic acid, present in some legumes
used as a popular meat substitute,
can also reduce the bioavailability
of nutrients and compounds present.
So, what may superficially appear
“the same”, has the potential to mask
important differences in nutritional
values. A 2019 consumer survey conducted by the International Food
Information Council highlighted how
presenting nutritional information
in this way can impact consumer
attitudes and opinions towards foods
that will inevitably influence their
food choices.
ARE PLANT-BASED FOODS NUTRITIONALLY EQUIVALENT TO
ANIMAL PRODUCTS?
In short, the answer to this question
is no. However, that’s not to say meat
= good, plants = bad, there are wins
and losses on both sides.
A 2021 study by Dr. Stephan van
Vliet and colleagues at Duke University School of Medicine, published
in Science Reports, highlighted some
of these discrepancies at the metabolic level. The team used untargeted
metabolomics to explore the metabolite profiles of grass-fed ground beef
and a popular plant-based alternative
that had been matched for serving
size and fat content. While the
nutritional labeling on the products
appeared very similar, their analyses
revealed around a 90% difference
in metabolite profile between the
foods that spanned the nutrient
classes, including amino acids, fatty
acids, vitamins and phenols. Teasing
these results apart showed that 22
metabolites were found exclusively
in the beef and a further 51 were in
greater quantities in the beef than the
plant-based alternative. However, 31
metabolites were found exclusively
in the plant-based alternative and a
further 67 were in greater quantities
than in the beef product. While the
beef provided compounds such as
glucosamine, which benefits connective tissues and blood vessels,
and antioxidants including squalene
that the plant-based product lacked,
the plant-based alternative provided
vitamin C and phenolic antioxidants
among other beneficial molecules
that were absent in the beef.
The study gives us a taste of just
how different these apparently
similar foods are and the problem
with routinely tracking (around 150
in the case of many databases) and
reporting (around 13 in the case of
most nutritional information labels)
only a fraction of the nutritional
components and/or grouping them
together. This also highlights that
studies based on label or categorization information rather than more
comprehensive analytical data may
miss important shortcomings and
benefits of both animal products and
their alternatives.
The authors concluded from their results that, on this basis, the products
shouldn’t be viewed as interchangeable but instead as complementary
to one another from a nutritional
standpoint. They write, “The complexity of the whole food matrix—as
indicated here by our metabolomics
findings—highlights that attempting
to mimic food sources using single
constituents such as isolated proteins, vitamins and minerals is challenging and arguably underestimates
the complexity of the food source it is
meant to mimic.”
This echoes evidence from other
groups that protein sources are not
metabolically or nutritionally equivalent, with De Marchi and colleagues
finding 21-fold less methionine—an
essential amino acid for humans—in
plant-based compared to beef burgers. They concluded that complete
and unbiased nutritional information
on such products is necessary so
that consumers can adjust their diet
accordingly to continue to meet their
nutritional needs. Since then, further
studies have gone on to add to this
body of evidence, look for alternative
sources that do deliver high-quality
protein and ways to bridge the gap.
A NEED FOR GUIDANCE
A number of studies have also highlighted nutritional disparities of animal, traditional plant-based and novel
plant-based foods that may be visible
on nutritional labels but to which many
of us may pay little attention based
13
on our preconceptions about plantbased alternatives compared to their
animal-based counterparts. In a bid to
enhance the sensory appeal of novel
alternative proteins over some traditional plant-based substitutes, some
products have turned to the addition of
salt, sugar and fat and are themselves
classed as ultra-processed foods.
A 2021 study from Tso and Forde
found that while a diet of traditional plant-based substitutes met daily
requirements for calcium, potassium,
magnesium, phosphorus, zinc, iron
and Vitamin B12 and was lower in saturated fat, sodium and sugar than the
reference omnivorous diet, diets based
on novel plant-based substitutes failed
to meet daily requirements for calcium,
potassium, magnesium, zinc and Vitamin B12 and exceeded the reference
diet for saturated fat, sodium and sugar.
A 2022 study from researchers at the
University of Göttingen highlighted
the differences in nutritional values
for both meat and cheese against
their plant-based alternatives using
nutritional scoring and analytical
chemistry-based techniques to evaluate vitamin and mineral content. The
work highlighted that fat, saturated
fatty acids and salt in particular varied
considerably between the samples
tested. While some of these differences may be visible on nutritional labels,
the authors suggested that because of
these types of differences, “consumers
need guidance on how to compose a
balanced plant-based diet.” This message is reinforced by a UK study highlighting the high level of salt in five out
of six plant-based meat alternatives
tested, with three quarters not meeting
UK salt intake guidelines.
In Canada, legislation has been introduced this year stipulating criteria for
the protein content, protein quality
and minimum content of a range of vitamins and minerals permitted in meat
alternatives to try and help address
some of these concerns.
FILLING THE GAP
Redressing nutritional inequities,
both present and absent from nutritional labels, between alternative
protein sources and the animal
products they are seeking to emulate
is understandably an active area for
future foods research. Enhancing the
micronutrient profile while reducing
reliance on nutritionally sensitive
components such as salt and sugar are
of particular interest.
Fortification is one method by which
missing components may be added
to meat alternatives. Vitamin B12
is a good example, being important
to health but typically absent or
only present in unavailable forms in
plants. However, adding individual
components without the complex
matrix in which they are found in
the original meat product may mean
that synergistic benefits gained
from meat consumption will still
be impaired.
Genes may be engineered in that
enhance the capabilities of plants or
cells to produce these missing nutrients. This approach has been utilized
successfully to enhance the nutritional value of bovine cells used to
produce cell-cultured meat. However,
in this particular case, the scientists
sought to provide added nutritional
benefits that would not normally be
present in the original meat product
rather than add back in something
that was missing.
Even if a nutrient is present in both
the meat and alternate protein source
on analysis, that does not necessarily
mean that its bioavailability is the
same in both foodstuffs. Take iron for
example. In meat, iron is present in
heme, which has greater bioavailability than the non-heme form found in
plants such as legumes that provide
popular meat alternatives. To address
this, scientists have developed a novel
plant-based alternate protein source
in which the iron is present in the heme
form thanks to a soy leghemoglobin
protein preparation derived from
Pichia pastoris yeast. However, while
encouraging, this is far from standard
practice in foods we are likely to find
on supermarket shelves.
FOOD FOR THOUGHT
While recent innovation in the alternative protein source space has seen
great improvements in organoleptic
properties such as texture, taste and
appearance, it would appear that there
is still much to be done to address nutritional inequalities. The general message
seems to be that alternative protein
sources, especially those derived from
plants, can provide a more sustainable
alternative to animal products but
currently there is a significant degree
of nutritional variability within the
field. Consequently, it is important that
consumers do not see many of these
products as a straight swap or consider
only the protein quantity of the product, with a more flexitarian approach to
their consumption advised by some. At
risk groups such as children, pregnant
women and the elderly need to be
particularly careful and improvements
in regulation and legislation standards
could help to safeguard shoppers. ⚫
It is important that consumers
do not see many of these
products as a straight swap
or consider only the protein
quantity of the product.
It’s simple, just choose the groups and
click the bubble.
Our Facebook groups allow you to keep up to
date with the latest news and research and share
content with like-minded professionals. With three
diverse groups there is something for everyone.
Hi. How are you? Have you seen our
Facebook groups?
Thanks! I’ll come join the conversation.
Come join the Neuroscience, News
and Research Group here.
Discover the Analytical Science
Network Group here.
Explore The Science Explorer Group here.
No I haven’t. Could you tell me more?
Sounds great! How do I join?
Biology
NATURE'S TOOLS, REDESIGNED
KERRY TAYLOR-SMITH
AnnaMaria Vasco
16
iStock Credit Line
S
ynthetic biology is a multidisciplinary field that remodels
organisms for useful purposes
by engineering them to have
novel abilities, often drawing inspiration from nature to build molecular
components that don’t exist in the
natural world.
It uses living organisms to solve problems by breaking them down into
individual components and applies
engineering principles to coordinate
changes to R NA, DNA and proteins
to form biological systems with
novel functions. These systems can
be designed based on known biology, or from scratch, to carry out a
certain task.
“I like a simple definition, that contrasts analytic biology, the study of
living systems that already exist in
nature, with synthetic biology, which
makes new or modified living systems that do not exist in nature,” says
Dr. Jamie A Davies, professor of
experimental anatomy at the University of Edinburgh. “This links nicely
with the dichotomy between analytical chemistry, the study of what
already is, and synthetic chemistry,
which makes something new.”
Synthetic biology has several useful applications, in conservation for
example, to restore biodiversity and
confer disease and stress resistance,
in bioremediation to clean pollutants
from the air and water, in manufacturing, agriculture and farming and
in biofuels.
In medicine, synthetic biology is
helping scientists to better understand diseases and treatment. It can
be used to produce drugs and new
vaccines, improve monitoring and
diagnosis and alter human cells with
properties designed to help patients.
Research is also turning its attention
to building new tissues.
SYNTHETIC BIOLOGY AND
THERAPEUTICS
Nature is an important source of bioactive products, and therefore often
a source of new therapeutics; over
the last 30 years most anticancer, anti-infective and anti-bacterial drugs
originated from natural sources, like
plants, fungi or bacteria, and their
derivatives. But developing a new
therapeutic is time-consuming, expensive and challenging; it can take
10 to 15 years from target identification to approval, and cost as much as
$2.9 billion per drug, with only 1 in
5,000 targets making it to market.
Synthetic biology can aid the design
of new therapeutics in two broad
ways, says Davies: “One is epitomized by CAR T cells used to treat
cancer: engineering human cells to
have new properties that are useful,
for example, the ability to mount a
ferocious attack on a patient's tumor,
and put them back into the body to
do that job.”
CAR T, or Chimeric Antigen Receptors T-Cell Therapy, is a highly complex and innovative type of immunotherapy that harnesses a patient’s
own immune system to target cancer
cells. T cells or lymphocytes are
harvested from the patient’s blood
and genetically engineered in the lab
to recognize and bind to specific proteins, or antigens, on the surface of
cancer cells, before eradicating them.
These therapies initially focused on
acute lymphoblastic leukemia (ALL),
the most common cancer in children,
but have the ability to eradicate very
16
iStock
17
advanced leukemias and lymphomas,
and can keep cancer at bay for several years after infusion.
The second way that synthetic
biology can aid drug discovery,
says Davies, is engineering cells to
perform the physiological role of
another, for example, non-pancreatic
cells to make insulin in response to
blood sugar. This application is currently experimental and not yet used
in humans.
“People with type 1 diabetes destroy
natural pancreatic cells because of
an autoimmune problem, but do not
destroy other cells, like skin cells,
so engineering these other cells
to do the insulin job would cure
diabetes,” he says. Scientists at the
J. Craig Venter Institute have engineered bacterial cells that naturally
live deep within the skin to function
like insulin cells, which entered
preclinical testing in 2021. “A nd
you can extend this idea to many
similar diseases,” Davies says.
The other broad way is using engineered metabolic pathways to produce difficult-to-make drugs, notes
Davies: “The real example in current
use is engineering production of the
antimalarial drug artemisinin into
yeast cells, by 'borrowing' genes
from plants to build up a new metabolic pathway in the yeast. A nd this
idea could be extended to all sorts
of other molecules.”
The main source of artemisinin is
extracted from Artemisia annua;
however, its artemisinin content
is only 0.1–1% – nowhere near
enough to meet global demand. The
chemical synthesis of artemisinin is
complicated, expensive and offers a
low yield, so the ability to produce
artemisinin in vivo using synthetic
biology is attractive.
Researchers have explored the
option of synthesizing artemisinin
using Escherichia coli (E. coli) and
Saccharomyces cerevisiae (S. cerevisiae) as chassis cells – a cellular host
or recipient of engineered biological
systems in synthetic biology – and
yeast as the cell factory, thus transferring the production of artemisinin
from plant to yeast.
Synthetic biology is highly and
rapidly adaptable, making it ideal
to respond to urgent needs like
vaccines. An approach focusing on
large-scale nucleic acid manipulation was successfully used to create
a COVID-19 vaccine, while genomic
codon deoptimization is used to
create injectable and nasal vaccines
against the f lu and respiratory
syncytial virus. Other vaccines are
based on introducing DNA and R NA
components encoding viruses into
human cells. These cells produce viral antigenic peptides in a repetition
of the natural infectious process to
induce immunity.
SYNTHETIC BIOLOGY AND
DIAGNOSTICS
Synthetic biology also has a role to
play in diagnosing disease; systems
can be introduced into cells to sense
biological signals and chemical
substances within the cell, and even
respond to stimuli.
Davies says synthetic biology’s role
here is to engineer cells or proteins
to be sensitive and accurate sensors
of markers of disease: “This might
include a simple test, a drop of blood
or urine, or even a patch on the skin,
to report all the time to a reader that
can talk to a mobile phone and let
doctors monitor how someone is doing. These sensors offer the promise
18
iStock
to be combinatorial too – to measure
many things at once.”
Techniques based on gene circuit
construction and rapid, iterative
prototyping have enabled the
development of devices, ranging
from whole-cell living assays
to engineered cell-free nucleic
acid sensors and amalgams in between, which have been applied to
non-communicable diseases such as
cancer and coronary artery disease,
and communicable diseases.
One area of active research is
paper-based toehold switch R NA
sensors. RNA toehold switch sensors are robust molecular switches
that can detect R NA molecules in
a sequence-specific way, and when
combined with a paper-based cellfree molecular diagnostic platform,
are capable of detecting Ebola, Zika
virus, COVID-19 and other respiratory diseases.
Another is the use of CR ISPR-Cas
technology as a rapid molecular
diagnostics tool for nucleic acids and
detection of pathogens. The technique enables researchers to target
specific stretches of genetic code
and to edit DNA at precise locations.
Each disease-causing virus or
microbial pathogen has a characteristic sequence of nucleic acids.
CR ISPR-Cas technology can recognize and snip this specific nucleic
acid sequence in a sample, meaning
it can be used as a diagnostic biomarker. Screening based on CRISPR-Cas can be helpful in searching
for biomarkers that identify populations sensitive to a certain cancer
treatments, for example.
SYNTHETIC BIOLOGY AND
AGRICULTURE
CR ISPR isn’t just limited to medical
applications; it also has a role to play
in farming and agriculture, where it
is an effective technique for manipulating a number of crops, including
maize, wheat and apples.
Genetic editing can be used to introduce desirable qualities into plants
in a faster, controlled and specific
way. It allows scientists to make very
precise changes to the plant’s DNA
to confer traits, such as immunity or
resistance against a certain pests or
pathogens, to change how the plant
grows or to change the soil microbiome to help improve crop yield
and quality.
“Synthetic biology approaches, like
genetic editing, can help improve
food security, increase the yield
and the resilience of crops in the
face of climate change,” says Professor Alistair McCormick, chair
of plant engineering biology at the
University of Edinburgh. “The
population is expected to reach
10 billion by 2050, and we need
to improve all of these to feed the
growing population.”
Genetic editing gives us the ability
to grow the same crops on the same
amount of land, but with a better
yield, says McCormick, citing the
recent example of knocking out the
gene KRN2 in rice and maize, which
led to 8 and 10% increases in grain
yields, using thanks to gene editing.
The technique also allows scientists
to alter the characteristics of staple
crops like rice, which have been
modified to produce beta carotene,
a nutrient typically associated with
preventing vitamin A deficiency ,
and to develop a type of wheat less
likely to produce acrylamide, a
potentially carcinogenic compound,
during cooking or baking. This
Synthetic biology is helping
scientists to better understand
diseases and treatments.
19
wheat has recently been subject to
its first ever field trial and showed a
significant reduction of acrylamide
when the f lour is baked.
Synthetic biology can also be used
to produce healthier or specifically
designed food ingredients, like
high-value proteins, lipids and vitamins. Food production by microbes
is considered a promising alternative that could allow food to be produced quickly in an environmentally
friendly way.
However, such techniques will
not be enough to meet the growing demand for food, McCormick
adds – increasing crop productivity is essential for global food
security. His work focuses on
photosynthesis and how it can be
manipulated to produce novel products or improve plant productivity.
McCormick’s group aims to improve
photosynthetic CO2 assimilation
by engineering algae to increase
photoassimilation rates and therefore productivity.
He notes that growing algae and
heterotrophic species together is
a “hot new research topic”. If these
species can be grown together, then
CO2 could be captured, converted
into sugar and then used by the cell
media. Researchers at the University of Michigan recently created
a strain of cyanobacteria that can
export up to 85% of the carbon it
fixes as sucrose. Their approach,
they say, “is promising as a potential alternative to land-based crops
for sugar production, as this level
of productivity could exceed sugar
output from sugarcane or corn if it
could be scaled up.”
Another “hot topic” is that of engineered probiotics designed to
improve health by increasing the
specificity of probiotic microbes or
improving the antimicrobial activity
of the probiotic against pathogenic
infections of the gastrointestinal
tract. Synthetic biology approaches
have been used to engineer the
probiotic bacteria, Lactobacillus
johnsonii (L. johnsonii) to be effective against Clostridium perfringens
(C. perfringens) a bacteria that
causes food poisoning.
SYNTHETIC BIOLOGY’S
IMPACT, NOW AND IN
THE FUTURE
Davies says synthetic biology's
greatest current strength is that it
allows scientists to test their understanding: “In the same way that you
can test your understanding of aerodynamics by making simple paper
planes, we can test our understanding of complex biology by making
simple things that work according
to the principles we have derived,
and we can see if they really work.”
“A nd when they don't, that tells
us we have not really understood
things at all and need to go back and
do better analytic science,” he adds.
Davies believes tools like CA R T
will continue to grow and will have
greater impact, as will non-medical
applications, like organisms engineered to clean up water, which are
important for health. “But most
of this is still very expensive and
experimental, and I think it will be
a while before we see real impacts,”
he says. “For now, the impacts are in
research itself.”
McCormick believes synthetic
biology will evolve into engineering biology – a move already being
championed by UK R I. He says
it will become more inclusive of
branches like bioengineering and
increase its remit to chemistry,
physics and material science. The
biggest impact, in his opinion,
will be “in high value products, in
pharma and in vaccines”.
“A nd it will make a big difference
in agriculture to ensure we get as
much as we can out of what we
have. It may not solve the problem,
but it will certainly help ease it,”
he concludes. ⚫
iStock
20
iStock
P
rofessor Donald Ingber, founding director of the Wyss
Institute for Biologically
Inspired Engineering at Harvard University, is responsible for
the development of human organ
chips. These organ chip systems
provide researchers with a window
into inter-organ physiology and the
body’s response to drugs, without
the need for animal testing.
These chips can be applied to drug
development, disease modeling and
personalized medicine.
Technology Networks invited Ingber
to an Ask Me Anything session to
answer your questions about this
incredible technology.
Lucy Lawrence (LL): What is a
body-on-a-chip system?
Donald Ingber (DI): So, we call
them organs-on-chips or organ chips,
and if you link them together, you can
get a body-on-a-chip. The organ chips
that we first developed were small devices the size of a computer memory
stick. They are optically clear and
made out of a f lexible polymer silicone rubber. They have two hollow
tiny channels less than a millimeter
wide that run parallel on top of each
other and are separated by a porous
membrane. We can put different cell
or tissue types on either side [of the
membrane] to create tissue-tissue
interfaces, which is why we call it
an organ-on-a-chip rather than a tissue-on-a-chip.
We are able to stretch and relax the
membrane and attached tissues
How Body-on-a-Chip Tech Is
Transforming Research
KATE ROBINSON & LUCY LAWRENCE
21
Technology Networks, adapted from Organs-on-Chips: Furthering Our Understanding of Disease
laterally, so we can mimic breathing
motions if we have air above. If we
have f luids on both sides, we can
mimic peristalsis or deformation in
any tissue.
We've been able to mimic physiology
and disease states, drug actions and
toxin actions with incredible fidelity.
If you flow medium through the
vascular channel line by endothelial
cells, through the chip, you could take
the effluent and put it into the inflow
part of the next chip, to create a bodyon-a-chip. This allows you to look at
multi-organ coupling.
LL: What sparked your interest in
body-on-a-chip technology and
organ-on-a-chip technology?
DI: When I was an undergraduate
student, I had insight that mechanical forces might be as important
for cell shape as chemicals and
genes, and that led me on a path to
experimental testing. Eventually, I
convinced people that it was true
by developing ways to control cell
shape via distortion or stretching. In
our first paper published in 1994, we
adapted computer microchip manufacturing techniques to gain control
over features at the same nanometer/
micrometer scale at which cells live.
We also created a technique to control how far cells can spread by making islands coated with extracellular
matrix the size of cells. That's how we
got into microchips and cell biology.
We then started making hollow channels that are called microf luidics
and then we began culturing cells in
those channels.
LL: What are the challenges or
limitations that you've come
across while developing and
using your body- and organ-ona-chip systems?
DI: With organ-on-a-chip, the biggest limitation we faced were bubbles. The tubes are very small, so, if
you get bubbles in them by connecting and disconnecting tubes, this
can cause the cells to die. In efforts
to commercialize organ-on-chip systems, “click and play” approaches are
being developed that don’t have connectors. This has made the technology much more robust, and has helped
enormously.
Cell sourcing is always a challenge,
whatever in vitro model you use,
we find that organoids and primary
adult cells have been the best over induced pluripotent stem cells because
they retain epigenetic signatures
and disease states.
R ight now, the chips are relatively
low throughput, but incredibly
high content.
Whole body-on-chips have additional problems, as you now have to use
multiple cell types for culturing and
you have to time them so that they
can be put on the chips, differentiated and all aligned so the chips can be
coupled together.
But developing noninvasive readouts, whether we use electrodes,
oxygen sensors or f luorescent
reporters, are challenges that you
would face in any culture system.
A nother challenge is the amount of
data generated, similar to an animal
model, and for people used to 96-
well plates with quick assays, it's
very different.
Figure 1: A three-layer microfluidic chip model with cells grown on a porous membrane.
"We can put different cell or
tissue types on either side [of
the membrane] to create tissuetissue interfaces, which is why
we call it an organ-on-a-chip
rather than a tissue-on-a-chip,"
says Ingber.
22
LL: Can organ chips be used
to study microbial infectious
diseases?
DI: Absolutely. Not only can we study
pathogens, but we can also study
healthy microbiome versus diseased
microbiome. Another major advantage of microfluidic organ chips as opposed to microphysiological systems
is the ability to keep a complex microbiome in direct contact with live
human cells for multiple days. If you
took an organoid and put microbes
on it, you would have about 16 to 24
hours before they die.
So, we've used diluted stool samples
from patients in the lumen of our intestine chips. If the microbiome is healthy,
they remain intact for five days. If it’s
unhealthy, you will see blunting of
the villi, compromised barriers and
inflammatory cytokines.
We recently published a paper on
a vagina chip with healthy microbiome versus disease, and we're using
the chip with the Gates Foundation
to test live biotherapeutic products.
These products for bacterial vaginosis are moving to clinical trials in
South Africa and the United States in
the next few months, and we can see
these actually suppress inflammation
on these chips.
In terms of pathogens, we've done
viral infection and bacterial infection
of intestine chips. We use metabolomics and can figure out which metabolites mediate the microbiome. In
this case, we were looking at human
microbiome protecting against enterohemorrhagic E. coli versus mouse
microbiome, because humans and
mice have totally different sensitivity.
LL: Could your replica models be
linked to an artificial intelligence
(AI) environment for studying
and testing?
DI: We have papers in preprint and
in development where we used machine learning and A I approaches.
In these studies, we started with
transcriptomic data from patients
to see which genes across the
whole transcriptome would need to
be “f lipped” to make faulty genes
healthy. We then looked through all
the available transcriptomic data on
the web for every known drug, and
then the AI machine learning computational tool prioritizes which
[drugs] are most likely to reverse
the state. These can then be explored more using in vitro models,
including organ chips, to get transcriptomic data on human models.
The interesting thing about the
computer tool is that it also analyzes known gene networks and
known gene drug networks, and
you can identify drug targets and
then not only repurpose existing
drugs, which we've done and moved
to clinical trials, but actually
identify new targets and identify new drugs.
We also do it the other way around,
by which we use a molecular dynamics simulation for drug molecular design, and then we integrate machine
learning and A I, in this process. We
use organ chips coupled with it to
generate human data. ⚫
Professor Donald Ingber was speaking to
Lucy Lawrence, Senior Digital Content
Producer for Technology Networks. Editing by Kate Robinson.
Prof. Ingber’s groundbreaking research
has transformed the
landscape of biomedical
science and opened new
avenues in drug discovery,
disease modeling and
personalized medicine.
23
iStock
hough tells me, believed that speech was
something that began as a purely social
instrument for communication between
people that over the course of development became gradually internalized. This
process of internalizing, Fernyhough
says, gives us “tools for thinking” that
benefit our development.
AN EVOLUTIONARY BENEFIT
TO INNER SPEECH?
Not all aspects of our inner speech give
obvious advantages to our behavior.
Anyone who has anxiously spent
hours internally processing worried
thoughts about an exam, only to have
no time to actually study for it, might
wonder why such unhelpful examples
of inner speech were not chopped out
at an earlier point in evolution. Surely
an early human would have been
much “fitter” to their environment if
they just threw a spear straight into
a mammoth without ruminating on
how they were going to extract the
spear later, and whether this particular
mammoth was going to be as delicious
as the one they had caught last winter?
Jonny Smallwood, a professor in the
Department of Psychology at the
University of York, has made the
study of one particularly aimless form
of rumination, daydreaming, his own
research niche. “Things like daydreaming, even though they might seem
“purposeless” must be having some
kind of quite important role in how
we guide our lives,” says Smallwood.
But what is that role? Smallwood’s
studies have looked at how people
from different countries and cultures
daydream. All his participants had one
thing in common – they tended to think
about the future. Smallwood reckons
that this common finding hints at why
internal states like daydreaming and inner speech have become so widespread.
“One of the ways that the internal
representation system can be selected
for is because you can prepare for an
interaction with another person and
you can think about the kind of things
that they might be happy or unhappy for
you to say. Then, when you get into that
circumstance, you’re less likely to say
the wrong thing, which might make the
interaction smoother,” says Smallwood.
NO SUCH THING AS A
UNIVERSAL BEHAVIOR
Internal processes like inner speech
and daydreaming might give us an
evolutionary advantage. But the most
interesting thing about these processes
isn’t their function, but their prevalence.
Fernyhough has noted that inner
speech, despite perhaps seeming to
many people like the most innate behavior of all, is not ubiquitous. “You certainly find that private speech in children is
pretty universal. You don’t find many
kids who are developing in a typical way
that don’t use private speech. But when
it comes to adults, I came across people
who clearly just weren’t doing much
inner speech,” says Fernyhough.
These internally silent volunteers
instead commonly relied on imagery in
their day-to-day thoughts, with pictures
replacing words as their thinking tool of
choice. “To my mind it says it’s something that a lot of humans do because it’s
handy. But it’s by no means an essential
component of consciousness,” says
Fernyhough. “We find different ways
to get to the same outcome and I think
that’s one of the marvels of psychology.”
Variation in how we think isn’t limited to
whether we use words or images. Sometimes, the very nature of our thinking
can become disrupted. Fernyhough
became acutely aware of this when he
shared his developmental psychology
findings with psychiatrist colleagues,
who took his comments about inner
speech to be referring to auditory hallucinations, or “hearing voices”.
These hallucinations are most commonly
linked in popular culture to the mental
health disorder schizophrenia. In reality,
schizophrenia is a complex disorder, and
auditory hallucinations are just part of an
often varied range of symptoms. The idea
that hearing voices is unique to schizophrenia is also misleading, suggests
Fernyhough. “The experience of hearing
voices is involved in all sorts of different
psychiatric diagnoses, everything from
post-traumatic stress disorder (PTSD)
to eating disorders. It is also experienced
by quite a small but significant number
of people who are not mentally ill who
hear voices quite regularly, but don’t
seek help for them because they’re not
troubled by them.”
HEARING THE VOICE
Is there a fundamental difference between
inner speech and auditory hallucinations?
This question has been the target of a
project Fernyhough is helping run at
Durham, funded by the Wellcome Trust,
called Hearing the Voice. The study is still
ongoing, but some early conclusions
are that the difference between these
internal states is very simple. “The idea
is that when somebody hears a voice,
what they’re actually doing is some inner
speech, but for some reason, they don’t
recognize that they themselves made that
bit of language in their heads,” says Fernyhough. “It’s experienced as coming from
somewhere else or from someone else.”
What complicates this idea are the many
types of both inner speech and auditory
hallucinations. Fernyhough thinks that
his theory will apply to some types of
both experiences, but not all. Some
hallucinations have acoustic properties,
as if the speaker is in the room with you.
Sometimes the voice has an accent or
a timbre or a pitch. “It’s very hard to pin
down what it is that makes some people
have an experience that feels alien, that is
distressing, especially when some people
have what seems to be the same experience, but don’t find it distressing,”
Variation in how
we think isn’t
limited to whether
we use words or
images. Sometimes,
the very nature of
our thinking can
become disrupted.
Disseminate Your
Research With Us
We know how important it is to disseminate your findings
when working in research, but also what a challenge it can be
to find opportunities beyond the conference hall. We want to
address this!
Technology Networks is spearheading
a project to help researchers raise
the profile of their work to a wider
audience and increase coverage of
the fantastic research papers out
there that may not get the media
attention they deserve.
There is a lot of great
research output and a very
engaged audience that wants
to hear about it, so share your
work with us!
Find Out
More
24
iStock
T
he Enabling Nutrient Symbioses in Agriculture (ENSA)
project seeks to develop cereals that carry the same ability
as legumes have, to biologically fix
nitrogen by harnessing interactions
with soil microbes. By reducing the
need for nitrogen fertilizer, the project could have a profound impact on
sustainability in agriculture.
In this interview for Technology
Networks, Professor Giles Oldroyd,
director of the Crop Science Centre
at the University of Cambridge
and leader of ENSA, discusses the
project’s research mission and the
landscape of food and agriculture
more generally, including the recent
passing of The Genetic Technology
(Precision Breeding Act) in the UK.
Molly Campbell (MC): Your research mission is to eradicate
the need for inorganic fertilisers
in agriculture through the use
of beneficial microbial associations. How are you working to
achieve this?
Giles Oldroyd (GO): The ENSA
project aims to deliver environmentally sustainable crop innovations
that boost the yields and livelihoods of smallholder farmers around
the world.
Rather than intensifying the use of
chemical inputs, such as inorganic
fertilizers that are both environmentally harmful and inaccessible
to many small-scale farmers, ENSA’s
work aims to maximize the beneficial
microbial associations between
plants, soil fungi and bacteria.
We are working to develop crops
that make better use of the nutrients
already present in the air and the
soil, using many of the processes that
plants themselves have evolved over
millions of years.
In particular, our work is focused
on interactions with nitrogen-fixing bacteria and with beneficial
fungi that expand the surface of the
root and help capture of nitrates,
phosphates and water to support
plant production.
For the former, we are aiming to
expand the nitrogen-fixation ability
– currently only present in legumes –
The Latest Frontiers in Agricultural
Science
MOLLY CAMPBELL
25
into cereal crops, while also improving
the efficiency of nitrogen-fixation in
legume crops like soybeans and cowpeas. Likewise, for interactions with
beneficial fungi, we are aiming to optimize the performance of arbuscular
mycorrhizal associations in crops to
facilitate phosphate, nitrate, water and
micronutrient capture.
MC: In your opinion, what are the
latest innovations in food and
agriculture research?
GO: Our work has uncovered that
many of the genes essential for the
interaction with nitrogen-fixing bacteria are also essential for the association with arbuscular mycorrhizal
fungi. This means a core genetic
framework for establishing N-fixation exists within crops such as cassava and cereals, to support arbuscular
mycorrhizal associations.
At the same time, our work has also
discovered that our target cereal
crops have inherited processes involved in forming symbiosis with
arbuscular mycorrhizal fungi, which
appears to be the evolutionarily-earliest beneficial microbial association
in plants. This means the symbiosis
signaling present in cereals shares
many similarities with the signaling
pathway for recognition of nitrogen-fixing rhizobia in legumes.
Moving forward, our goal is to
re-network these pre-existing processes in cereal crops, rather than
creating new ones from scratch. If
successful, these approaches would
not only revolutionize small-holder farmer production but would
also deliver sustainable and secure food production systems for
high-income countries.
Other research opportunities that I
feel are very likely to have significant
impact in food production are around
the attempts to enhance photosynthetic efficiency, driving up the effectiveness of carbon capture and the
utilization of knowledge from mechanisms of plant immunity that are
creating opportunities to drive resilience to pathogens. And of course,
existing technologies – including Bt –
that are already very effective at controlling insect pests.
These traits have significant potential to stack together, alongside
our work in ENSA, which could
be revolutionizing with regards to
sustainable productivity.
MC: The Genetic Technology
(Precision Breeding) Act was
passed in the UK earlier this
year. Can you describe what this
means for researchers and commercial companies developing
gene-edited products?
GO: The Genetic Technology (Precision Breeding) Act has come at a time
of rising challenges to food systems
and food production, both globally
and in the UK itself. As background
context to the passage of the Act, the
UK imports around half the food it
consumes from more than 180 countries, many of which face rising temperatures, more frequent droughts,
degraded soils and new pest threats.
As the impact of climate change accelerates and creates new strains on
food production, farmers at home
and overseas can no longer afford
to rely on and wait for hardier crops
produced by conventional breeding
alone, which is unlikely to keep up
with the rapidly changing challenges
of new growing conditions.
iStock
NITROGEN FIXATION
Nitrogen fixation is a
biological process where
atmospheric nitrogen is
converted into ammonia
by microorganisms such
as bacteria and archaea.
Nitrogen-fixing bacteria
can live freely in soil or
within legumes, where
they produce ammonia that
helps the plant to grow.
ARBUSCULAR MYCORRHIZAL ASSOCIATIONS
The most common type of symbiotic relationship in plants and
microbes, mycorrhizal associations help to improve plant stress
resistance, nutrition, soil structure and fertility.
BT TECHNOLOGY
The bacterium Bacillus
thuringiensis possesses
genes that encode the
production of toxins.
These genes, when incorporated into transgenic
crops, help to promote pest
resistance. Bt corn, for example, has been
approved for cultivation
and commercialization in
some countries across
the world.
26
Having secured passage in March
2023, the Act opens up new possibilities for science and innovation that
could transform the pace of agricultural research and development, to the
benefit of the entire global crop science sector.
The law, first and foremost, allows
for the commercial development of
gene-edited food in England, accelerating what might otherwise be possible through natural processes and
conventional breeding over a longer
timeframe. This means researchers can
cut down the time it takes to supply
farmers with more resilient or higher
yielding crop varieties, which could be
the difference between failed harvests
and food shortages or food security
and continuous supply.
Likewise, the Act also clears the way
for a more diverse plant science sector
in the UK by mainstreaming genetic
technologies in a way that encourages
more research among public sector institutes and start-ups.
Finally, the Act also stands to generate new varieties, products and
technologies that can be adapted and
used by other parts of the world, including developing countries where
agriculture is a primary economic driver yet faces greater threats
from the more pressing impact of
climate change.
The act does not change the position
on genetically modified crops, which,
in the UK, like the EU, holds a very
restrictive position on release of GM
foods. While there are many important traits that can be delivered in food
crops through gene editing, there remain some traits, such as the transfer
of nitrogen-fixation, that require genetic modification. Hence, while I very
much welcome to advances brought to
bear by the precision breeding bill, I
believe we still need to consider how
we facilitate delivery of some of the
transformative traits that only genetic
modification will allow.
MC: Can you discuss the importance of creating sustainable
agricultural practices, why it can
be challenging and how we can
do better on this front?
GO: The input-heavy processes currently used by farmers, particularly
in high-income countries, are unsustainable and expensive. The Green
Revolution use of inorganic fertilizers promoted higher yields, but
at a significant cost to the environment. However, in many low-income
countries, fertilizers continue to be
inaccessible for the vast majority of
small-scale farmers, and agricultural
yields remain low as a result.
For instance, the synthetic N fertilizer supply chain was responsible for
estimated emissions of 1.13 GtCO2e
(gigatonnes of carbon dioxide equivalent) in 2018 alone, representing
10.6% of agricultural emissions and
2.1% of global greenhouse gas emissions. The use of inorganic fertilizers
also contributes to eutrophication
and biodiversity declines.
At the same time, most smallholders in
sub-Saharan Africa lack the resources
to buy inorganic fertilizers. For example, average nitrogen application rates
are 25 kg/ha in sub-Saharan Africa,
compared to 100 kg/ha in the USA (on
average). As a result, most smallholder
farmers are often working with very nutrient depleted soils, resulting in limited
yields. Average maize productivity in
sub-Saharan Africa is two tonnes/ha, as
compared to nine tonnes/ha in the USA.
iStock
27
iStock
There is therefore an urgent need
to reach similar yield gains as those
achieved from the Green Revolution,
but without the need for environmentally damaging and economically
unsustainable inorganic fertilisers.
MC: What should the key priorities be for food and agricultural
researchers in the near future?
GO: During my research career I
have observed a revolution in our understanding of how plants function.
This has been underpinned by technological advances in genetics and genomics. We are in a radically different
position in our understanding of biological systems than we were when I
first started working in a laboratory
30 years ago.
We have the opportunity to apply
this deep knowledge for the advancement of our food production systems,
creating more productive and far
more sustainable crops, in forms that
should be readily available to even
the world’s poorest farmers. While
it is important to continue to deepen
our understanding of how plants function, I believe it is equally as important to ensure we apply this knowledge to the betterment of society, in
this case, to more productive, more
sustainable and more equitable food
production systems.
Too often these more strategic approaches come secondary to discovery science. I argue the two should
go hand in hand and I personally find
it hugely motivating to think that I
could contribute to delivering impact. We face phenomenal challenges over the following decades, we
need plant science now more than
ever, but we also need plant scientists to apply their passion and intelligence to delivering future-proofed
food production. ⚫
Professor Giles Oldroyd was speaking
to Molly Campbell, Senior Science
Writer at Technology Networks.
“Genetically modified organisms
refer to crops where DNA that does
not originate from that species
remains in the final product. This
is distinct from gene-edited crops,
where tools such as CRISPR
or TALENs modify the existing
genome in the plant, without the
need for new genetic material
remaining in the final product,”
says Oldroyd.
ISSUE 04, JULY 2021
cy in
rain:
of
ology
ISSUE 06, SEPTEMBER 2021
Molecules,
Mountains and
Making the World
a Better Place
Regulating Heavy
Metals in Baby Food
#PostItNotePhD
The Alpha and Omega
of COVID-19: Yes,
the Pandemic Will
End (but Not Soon)
ISSUE 08, NOVEMBER 2021
Sustainable
Science
and the
Road to
Net Zero
Uncovering Key
Interactions
Between CancerDriving Proteins
Addressing
Disparities in
Healthcare and
Clinical Research
Closing the
Vaccine Gap
ISSUE 03, JUNE 2021
s a
HaulerISSUE 01, APRIL 2021
The Physicality
ofConsciousnessISSUE 07, OCTOBER 2021
Return From
Extinction
The Neuroscience
of Creativity
Hidden Secrets of the
Human Microbiome
COVID-19: Vaccine
Stockpiling
ISSUE 10, JANUARY 2022
What the World’s
First Pig to Human
Heart Transplant
Could Mean for the
Future of Transplants
Unpicking the
Complexities of the
Cancer Microbiome
A New Approach to
Treating Superbugs
Influenza and
the Holy Grail
Vaccine
ISSUE 09, DECEMBER 2021
Lost Women
of Science
Why the Meat
Paradox Causes
Cognitive Dissonance
for Millions of People
The Omicron Variant
Highlights the Need
for Smarter, FutureProof Vaccine Design
The Pursuit
ofGlobal,
Sustainable and
Cooperative
Open Science
ISSUE 02, MAY 2021
Biodegradation of
Synthetic Plastic in
the Marine Habitat
A Step Closer to
Orally-Delivered
Insulin for Diabetes
ThreePsychologyHistory, Mystery
and DNA Analysis
ISSUE 05, AUGUST 2021
Mental Health and
Mental Illness in
Higher Education
Tapping the Ancient
Power of Microalgae
Turning On the
Vaccine Tap
All
Cancers,
Great and
Small
Pio cae publicae, ad rem deffre, cre
meripie ntimus se nossoltum inclutum
esulabe mnihil te nos vatudes, unter
Download CTA
Make sure you never miss an issue
of The Scientific Observer.
Subscribe Now
Download the Magazine for FREE Now!