The Landscape of Agricultural Biotechnology
Nourishing our growing population in the face of climate change and diminishing resources will not be an easy feat.
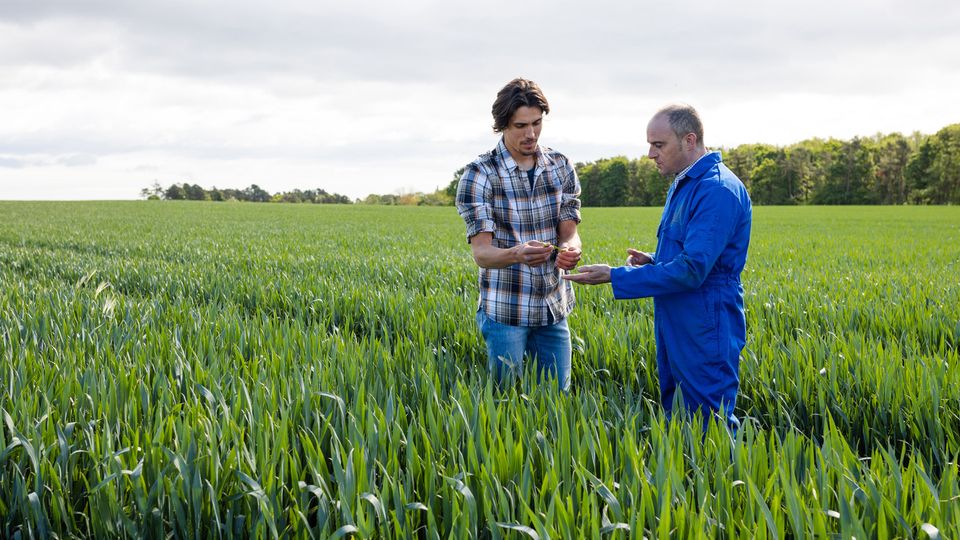
Complete the form below to unlock access to ALL audio articles.
By 2049, our global population will reach ~9 billion people. Pests, diseases and adverse environmental conditions are impacting crops across the globe, compounding the issue of feeding a growing population.
Traditional breeding techniques have enabled scientists and farmers to develop many varieties of plants and livestock tailored for specific agricultural or commercial purposes. Technologies such as genomic sequencing are helping to enhance these methods further. However, traditional breeding techniques can still take years to produce results.
Genetic engineering and gene-editing tools are by no means a panacea for the time-sensitive agricultural and food-related challenges we face – but they could help, if given the opportunity.
Genetic engineering
The invention of recombinant-DNA technology in the 1970s provided a new method for introducing desirable traits into a crop plant using genetic engineering. “A transgenic organism contains one or more genes that have been incorporated from another species, or across (trans) species, hence ‘trans-gene’,” Dr. Kevin Pixley, Dryland Crops Program director and Wheat Program director at the International Maize and Wheat Improvement Center, describes.
Genetically-modified organism – or GMO – has become the common term used to refer to transgenic organisms. GMO is not generally used to refer to organisms developed using selective breeding. Instead, it “refers to crops where DNA that does not originate from that species remains in the final product,” explains Professor Giles Oldroyd, director of the Crop Science Centre at the University of Cambridge.
A variety of laboratory techniques can be utilized to create transgenic crops. A simplistic overview of the process is as follows:
- Scientists decide which trait they want to insert into a plant and identify an organism that possesses the gene encoding that trait.
- The gene is isolated and copied, typically using polymerase chain reaction (PCR).
- To insert the gene into the organism’s cells, a vector is required that can carry the gene into the plant’s cells, such as a plasmid.
- The plasmid is introduced to the host plant’s cells, either using agrobacterium-based methods or biolistics.
- Once the gene is inserted, the cells are cultured in a laboratory and those that have successfully incorporated the gene are utilized to grow plants.
Examples of genetic engineering in agriculture
The first genetically engineered food product to be made available commercially was Calgene’s FLAVR SAVR™ tomato in 1994. The FLAVR SAVR™ was engineered to possess reduced polygalacturonase activity, an enzyme that dissolves pectin in the cell walls and causes the fruit to soften.
Over the last 25 years, transgenic crop production has undergone an over 100-fold increase; by 2013, over 4 billion acres of crops had been grown across 27 countries. These crops are typically classified by generation, where first-generation traits offer herbicide tolerance, resistance to pests or environmental conditions, and second-generation traits improve nutritional quality. Third-generation qualities are for which the applications “extend beyond those of traditional food items.”
Over 90% of US corn – the most commonly grown crop in the country – is transgenic, and an example of a first-generation trait. Corn growers are confronted by numerous pests, with lepidopteran larvae considered one of the most damaging. Bt corn is engineered to express genes from the soil bacterium Bacillus thuringiensis to produce Bt delta endotoxin, which kills the larvae. The selectivity of the protein against lepidoptera means that it is generally not harmful to other insects and is regarded as safe for humans and other mammal species.
Ninety five percent of canola crops – a staple ingredient in many cooking oils or margarines, and a commonly used animal feed – are transgenic in Canada. The crop is engineered to possess tolerance against ingredients found in widely used herbicides, which ensures that the plant can be sprayed and protected against weeds without experiencing damage itself.
Vitamin A deficiency, which can cause blindness or even death, is a major public health issue that affects 250 million people worldwide. Scientists engineered a combination of transgenes into rice that results in an increased production of beta-carotene, a precursor to vitamin A. “Golden rice” (GR) has been approved for cultivation in the Philippines, where a recent study found it could provide 57%–99% of the average vitamin A requirement for preschool children.
Transgenic crops could also support climate change mitigation. A 2022 study suggests that growing transgenic crops in the European Union (EU) could reduce greenhouse gas emissions by 33 million tons of CO2 equivalents per year, equivalent to 7.5% of the total agricultural greenhouse gas emissions produced in the EU in 2017.
Regulation of transgenic crops around the world
Many transgenic crops with diverse traits have been produced in laboratory settings, but ultimately the number of new, commercially available crops has been limited by a complex interplay of factors.
Transgenic crops cannot be grown or commercialized without approval from an appropriate regulatory agency, processes that ensure their safety both for human consumption and the environment. Legislation protocols vary across the world, with some regulations focusing on the product and others on the process, creating disparity.
In the US, three federal agencies are responsible for transgenics regulation: the Environmental Protection Agency (EPA), the Food and Drug Administration (FDA) and the United States Department of Agriculture (USDA). Recently, the USDA changed its position on assessing environmental risks from transgenic crops. Developers are no longer required to invest in intensive risk assessment if there is no scientific reason to believe that the crop is likely to cause environmental harm. An anthocyanin-rich purple tomato, developed at the John Innes Centre in Norwich, was the first transgenic approved under the USDA’s novel framework. Tomatoes carry the genes to produce anthocyanins, but they aren’t “switched on”. Researchers engineered the tomatoes to express two genes from snapdragons that work as genetic “switches”, helping to ramp up production of the antioxidant. “The bittersweet thing is that the tomatoes will be on sale in America and not the UK as well,” says Professor Cathie Martin, who developed the tomato in 2008.
That’s because the landscape is very different across the pond, and, despite leaving the EU in 2020, the UK currently adopts its regulatory regime when it comes to transgenic crops. “The EU has had a de-facto ban on almost all cultivation of transgenic crops for over 20 years,” Dr. Emma Kovak, senior food and agriculture analyst at The Breakthrough Institute explains.
The EU’s regulation of transgenics is considered an example of a process-orientated regulatory scheme. It adopts a precautionary approach to regulating transgenic crop cultivation and commercialization, where decisions are made on a case-by-case basis and require extensive scientific and safety trials. From 1992 to 2016, the EU had approved 2,404 experimental transgenic field trials for research, a stark contrast to the 18,381 approved in the US. Only 2 cultivation applications have been approved in the last 25 years in the EU, and critics have argued that achieving its requirements is so challenging that it likely deters researchers from trying.
Even if authorization for cultivation is made at the EU level, individual member states retain the right to ban cultivation in their territory. Cultivation of MON 810 maize, for example, has been banned by several countries including Germany.
The general public is also offered consultation on approvals, which can influence regulatory decisions. Public acceptance of transgenics has been notoriously turbulent – with a lot of skepticism deriving from “the publication of fraudulent, poorly designed and biased studies”, according to a recent article by Kathleen L. Hefferon and Henry I. Miller.
Elsewhere in the world, we are continuing to witness a growing eagerness for the adoption of agricultural biotechnology and the streamlining of regulations.
In Africa – where farmers are arguably most exposed to pressing climate, pest and disease challenges – there have been significant developments, says Oldroyd: “For instance, Nigeria approved pod-borer resistant cowpea, the world’s first transgenic cowpea, for commercial use in 2019. Likewise, amid historic droughts in 2022, the government of Kenya lifted a 10-year ban on the cultivation, as well as importation, of transgenic crops and animal feed, a move that is currently subject to an ongoing court case.”
Thorough scientific assessment of transgenic crops is necessary, but particularly strict methods can limit the development of scientific expertise, hinder innovation and have adverse economic and environmental impacts. In a 2021 opinion piece, Wu et al. argue that delaying the uptake of transgenic products that demonstrate clear benefits “has and will cost numerous lives, frequently of the most vulnerable individuals.”
“We need to feed people properly without destroying the planet,” says Professor Jonathan Jones, group leader at The Sainsbury Laboratory in Norwich. […] “Using GM methods, we can replace chemistry with genetics for pest and disease control.”
“The lesson from countries that have used this technology for 30 years is that its potential risks can be regulated on the basis that they are predictable and specific to the change being made,” Jones adds.
Gene editing
Gene editing allows scientists to modify gene sequences directly in an organism’s genome, a process that can introduce changes much faster than conventional breeding permits. Most gene-editing processes do not result in the introduction of DNA from a different organism.
“Advantages of genome editing are that these changes are precisely targeted and can be made within already excellent plant varieties, improving one trait, such as disease resistance, without altering any other traits,” says Pixley. “This contrasts with most breeding techniques, where mating a disease-resistant parent with another excellent parent produces progeny with half of the traits from each parent.”
A variety of genome-editing technologies now exist, such as zinc finger nucleases (ZFNs), homing endonucleases or meganucleases (HEs), transcriptional activator-like effector nucleases (TALENs) and the recent Nobel-prize winning CRISPR/Cas nuclease system. While their molecular components differ, these approaches all generate double-strand breaks in the DNA, activating the cell’s endogenous DNA repair pathway.
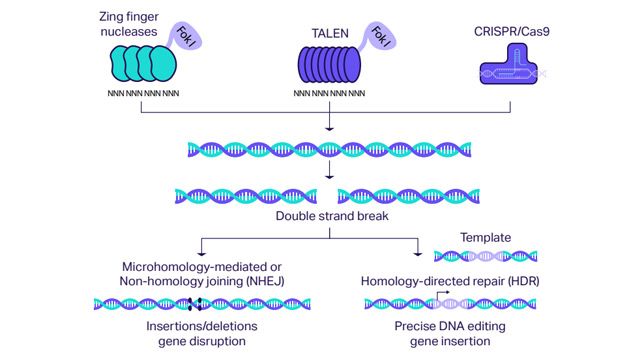
Figure 1: How CRISPR/Cas9 technology works. Credit: Technology Networks.
There are three types of edits that can be made, referred to as site-directed nucleases: SDN1, SDN2 and SDN3. Pixley describes SDN1 as the “simplest type of edit”, where DNA is cut and the natural repair mechanism results in the editing of only a few nucleotides at a precise location, altering the performance of a gene. “SDN2 is similar to SDN1, but a ‘template’ is provided, such that the reassembly of the DNA is not random,” he explains. “SDN3 is the most complex and involves the insertion of a complete gene or genes, often resulting in a transgenic, but differing from other transgenic plants in that the transgene is precisely inserted into the DNA at a carefully chosen site.”
Applications of gene editing
The number of research studies applying gene editing to plants continues to grow. The versatility, low cost and high efficiency of CRISPR and its derivatives has led it to take center stage in this space.
CRISPR is being used to develop a wide range of products that possess beneficial agronomic traits, consumer-focused traits and traits that are favorable for improving the sustainability of agricultural practices.
Rice supplies 20% of global calories but is particularly sensitive to drought in comparison to other cereal crops. Karavolias et al. used CRISPR-Cas9 to create stomagen and epidermal patterning factor-like10 (epfl10) knockout rice lines to fine-tune stomatal density, as previous research had demonstrated a reduction in stomatal density contributed to drought adaptation. Modest reductions in stomatal density were observed in epfl10 lines, without adverse reductions in stomatal conductions, carbon assimilation or thermoregulation. “These attributes could contribute to improved climate resilience in current and future conditions where water is limiting, and temperatures are increased,” the researchers say.
Rice is also susceptible to a disease known as bacterial blight, which can cause severe crop loss, particularly in Asia and Africa. Oliva et al. used CRISPR-Cas9 to introduce mutations into three SWEET gene promoters in rice lines. These promoters are required for the crop’s susceptibility to the pathogen Xanthomonas oryzae, which causes bacterial blight of rice. In paddy trials, rice containing genome-edited SWEET promoters showed broad-spectrum and robust resistance.
Other agronomic traits introduced using CRISPR technology include fungal, viral and temperature resistance and increased crop yield. Examples of consumer-focused traits include reduced browning, improved shelf-life, reductions in allergens and traits that address broader health issues. Raffan et al. recently published the results of Europe’s first CRISPR field trial, where wheat lines had been generated with the asparagine synthetase gene TaASN2 knocked out. “The aim of knocking out TaASN2 was to reduce the concentration of free asparagine in the grain. Free asparagine is converted to the carcinogenic processing contaminant, acrylamide, during high-temperature processing, baking and toasting,” the researchers describe. The gene-edited wheat lines had approximately 50% the amount of free asparagine as unaltered plants.
Gene-edited crops: The regulatory landscape
While most gene-edited plant varieties created with SDN1 or SDN2 edits do not contain genetic material from another organism, there is ongoing debate surrounding the biological, political, social and legal distinctions between gene-edited crops and transgenic crops.
A growing number of countries are updating their regulatory frameworks, making it easier to approve gene-edited crops for cultivation compared to transgenic crops. “Argentina was the first country worldwide to update regulations to make it easier, and many countries followed – including the US, Brazil, Canada, Paraguay, Ecuador, Chile, Colombia, Japan, Australia and Israel – with others considering such regulations,” Kovak explains.
These frameworks, says Pixley, largely consider SDN1 and SDN2 as “no different” from conventionally bred plants.
In 2018, the EU’s Court of Justice determined that gene-edited crops are required to meet the same regulation as transgenic crops. In July, the executive body of the EU – the European Commission – proposed a revision to these restrictions, which splits new genomic technique (NGT) plants into two categories. “Those that could occur naturally by conventional breeding, including those developed from gene-editing technologies that do not introduce foreign DNA, would be exempted from transgenic legislation and labeling requirements,” explains Oldroyd. “Conversely, all other NGT plants would continue to be treated as GMOs and will continue to require risk assessments and other authorizations.”
In the UK, a “game-changing” Act was passed into law earlier in March, which allows for the commercial development of gene-edited plants and crops in England. “This means researchers can cut down the time it takes to supply farmers with more resilient or higher yielding crop varieties, which could be the difference between failed harvests and food shortages – or food security and continuous supply,” says Oldroyd. The Act will help the plant sector mainstream genetic technologies to encourage further research by public sector institutes and start-ups.
“It also stands to generate new varieties, products and technologies that can be adapted and used by other parts of the world, including developing countries,” Oldroyd adds.
The UK government is phasing in the new framework, which does not affect the country’s stance on transgenic crops. “While there are many important traits that can be delivered in food crops through gene editing, there remain some traits, such as the transfer of nitrogen-fixation, that require transgenics,” Oldroyd says.
Access is a further barrier to wider use of gene editing in agriculture, says Pixley: “Some [technology] is protected by intellectual property rights, and [there are] trade barriers from potential importing countries that decide not to accept grains or plant products from genome-edited plants.” A 2020 study found that 1,232 out of 7,427 patent families relating to CRISPR were specific to plant modification. Patents can hinder research progress, especially for public sector or small-scale breeders, as licensing fees charged by companies that hold them might not be affordable for smaller organizations.
Innovation for a better world
The current landscape of agricultural biotechnology shows us that nourishing our growing population in the face of climate change and diminishing resources will not be an easy feat. Pixley emphasizes that while navigating these issues, there will be differences of opinion about the best approaches to follow: “But most of us will agree that food and nutrition security must be a basic human right for all – forever.” This, he says, is a great starting point to discuss science and innovation for a better world.
About the interviewees:
Dr. Kevin Pixley is the Dryland Crops Program director and Wheat Program director at the International Maize and Wheat Improvement Center. His research focuses on the use of crop biodiversity to address novel opportunities, including enhanced sustainability of farming systems, improved nutritional or health outcomes or value-addition for farmers.
Professor Giles Oldroyd is the director of the Crop Science Centre at the University of Cambridge. His research focuses on the interactions between plants and beneficial micro-organisms, both bacteria and fungi, that aid in the uptake of nutrients from the environment, especially nitrogen and phosphorus.