Techniques for Oligonucleotide Analysis
Whitepaper
Published: January 8, 2024
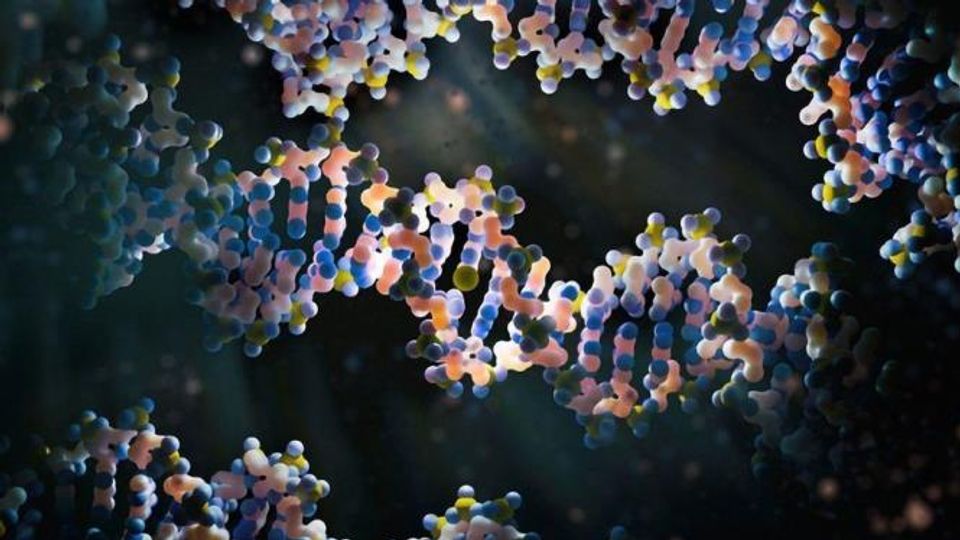
Oligonucleotides (short fragments of DNA or RNA) are an important resource for diagnostics and therapeutics. But in order for them to be clinically useful without adverse side effects, their synthesis and characterization must be rigorous.
Despite the increasing use of oligonucleotides in biomedical settings, their purification and characterization of their structural properties remain challenging.
This article presents current techniques in oligonucleotide production and characterization, highlighting recent advancements that enhance the efficiency of these methods
Download this article to learn more about:
- Common impurities introduced during oligonucleotide synthesis.
- Oligonucleotide purification and mass characterization techniques.
- Techniques to resolve oligonucleotide structures.
Techniques for Oligonucleotide Analysis
Article Published: October 27, 2020 | Andy Tay, PhD
Oligonucleotides, including deoxyribonucleic acid (DNA) and ribonucleic acid (RNA),
are increasingly being used in diagnostics and therapeutics. For instance, DNA can
be introduced into immune cells to genetically engineer them to express chimeric
antigen receptor proteins for cell-based immunotherapy.1 They are also being
used as DNA origami to mimic viral particles for designing molecular vaccines.2 A
variety of RNA, including messenger RNA (mRNA) and small interfering RNA
(siRNA), are also being used for transient expressions of proteins and interfering
with protein expressions respectively for therapeutic applications.
The need for high purity oligonucleotides
According to Balasubrahmanyam Addepalli, research associate professor at the
University of Cincinnati, despite the increasing use of oligonucleotides, it is still
challenging to purify them due to "failure sequences and final products with intact
protective groups."
The synthesis of oligonucleotides using techniques like solid-state synthesis can
introduce tiny amounts of impurities at each step of the synthesis cycle. As the
length of the oligonucleotide increases, the yield of the pure product decreases.
Modifications to oligonucleotides – such as attachment of polyethylene glycol to
enhance stability and bioavailability – can also introduce impurities during the
synthesis process.
The more common impurities are:
n-1 shortmers due to reaction failures
•
n+1 longmers due to single coupling step by successive addition of two
molecules of phosphoramidite and
•
Residual amount of phosphodiester from incomplete sulfurization and side
reaction.
•
A review by Goodchild eloquently summarizes the established technique for
oligonucleotide synthesis which has been automated since the 1970s.3 As the use
of oligonucleotides increases, it becomes increasingly important to adopt efficient
methods to purify, analyze and characterize them.
Purification of oligonucleotides
Polyacrylamide gel electrophoresis (PAGE) is a standard method that can be used
to separate oligonucleotides based on their size.4 Polyacrylamide gels are first
formed by the polymerization of acrylamide in the presence of a cross-linking
agent, resulting in a mesh-like gel network. Electrical fields are then applied,
causing migration of negatively-charged oligonucleotides to move towards the
positive electrode. Depending on the size of the oligonucleotide, the smaller
molecules move through the gel network more easily and quickly than their larger
counterparts. After a set amount of time, oligonucleotides of different sizes would
have migrated different distances in the polyacrylamide gel, allowing them to be
extracted and purified based on size.
However, PAGE offers low separation resolution if the oligonucleotides are similar
in size, with some modifications even being inseparable. Furthermore, PAGE
separation is laborious and time-consuming. These limitations have motivated the
switch from PAGE to liquid chromatographic (LC) approaches for oligonucleotide
purification.5
There are a variety of LC approaches for purification of oligonucleotides. For
instance, ion exchange LC separates ions and charged molecules such as
oligonucleotides based on their affinity to the ion exchanger. However, the
structures i.e. secondary folding, tertiary folding, dimers and aggregates of
oligonucleotides must first be denatured with organic solvents, high pH buffers or
high temperature (55-65 oC).
The most popular technique to purify oligonucleotides is ion-paired reversedphase
high-performance (IP RP HP) LC.6 In this technique, long-chained alkyl amine
is added at low concentration where it binds to negatively-charged
oligonucleotides in the mobile phase of LC. The retention and elution of the
oligonucleotides in the LC column are affected by factors such as charge of
oligonucleotides and length of alkyl chain in the ion pairing reagent such as
triethylammonium acetate. For instance, retention time generally increases in
proportion to the charges of oligonucleotides and hydrophobicity of the long alkyl
chain in the ion-paring reagent. Typically, researchers have to optimize parameters
such as column length, mobile phase flow rate, separation temperature and ion
pairing reagent buffer composition for oligonucleotide separation and
purification.7 A key advantage of IP RP HPLC is that it can also be coupled to a mass
spectrometer directly for detailed mass characterization of oligonucleotides.
Mass characterization of oligonucleotides
A way to analyze the purity of oligonucleotides is to analyze their mass using mass
spectrometry (MS). One of the common methods is matrix-assisted laser
desorption/ionization-time of flight (MALDI TOF) MS.8 This technique uses laser
light with a chemical matrix to ionize the oligonucleotide sample before
accelerating the ions through a flight tube to the detector which measures particle
counts as a function of time. The TOF is directly proportional to the mass of the
molecule. MALDI TOF MS offers high throughput and is ideally used to analyze
oligonucleotides below 50 bases, as the ionization efficiency and separation
resolution decreases. There is also a risk that modified oligonucleotides which are
photosensitive may be damaged by the strong laser source.
To overcome the limitation of MS, scientists are also combining techniques. Kimura
and colleagues recently combined deep sequencing and MS to characterize
transfer RNA (t)RNA.9 “Deep sequencing is a high-throughput method that reads an
incredible number of RNA sequences in a single run. However, deep sequencing
cannot identify the chemical properties of predicted modifications. In contrast, MS
analyzes the composition and position of modifications of oligonucleotides. tRNA is
the most heavily modified RNA molecules and historically, MS is used to identify
their modification profiles. Thus, in our paper, we took the benefit of both
analyses: taking deep sequencing for predicting modification sites and conducting
MS analysis for detailed characterization of the predicted modified sites,” says
Kimura.
The other popular method is electro-spray ionization (ESI) MS. This technique
applies high voltage to generate aerosol from liquid samples, ionizing target
molecules into multiple charge states represented by different mass spectra that
can be deconvoluted into parent peaks to identify oligonucleotides and potential
impurity. ESI MS is an excellent tool to analyze oligonucleotides greater than 50
bases and as it uses milder ionization condition, it is suitable for oligonucleotides
which are photosensitive. Nevertheless, it has lower throughput than MALDI TOF
MS. Therefore, depending on how urgent the characterization is and the tolerance
for error, either of these methods can be used.
Structural characterization of oligonucleotides
The most powerful way to resolve the structure of oligonucleotide is using X-ray
crystallography which has been indirectly linked to many Nobel prizes.10 X-rays
have a wavelength in the same dimensions as interatomic bonds in molecules of
about 1.5 angstrom and can provide highly-detailed structures of oligonucleotides.
Through analyzing the scattered X-rays, the electron density distribution in the
sample can be determined to reconstruct the internal molecular arrangement.
Nevertheless, reconstruction is not always easy due to loss of phase information
which has to be resolved using computational methods like Fourier maps. To
overcome the problem of loss of phase information, methods to synthesize
nucleoside and oligonucleotide analogs with selenium have been developed.11
These methods make use of the heavy atom, selenium, as an anomalous scattering
center or reference to enable phase determination.
Another major problem in sample preparation during X-ray crystallography is to
prepare the crystalized structures which require great care and is time-consuming.
To enhance the probability of crystallization, racemates have been added to
increase the number of molecular contacts.12 Racemic crystal structures of various
DNA sequences and folded conformations, including duplexes and quadruplexes
have even been demonstrated to be suitable for structure elucidation. RNA
crystallography is especially challenging due to paucity of surface chemical
diversity and poor flexibility- not ideal for crystal packing. To address this issue,
Sherman and colleagues created the Fab (fragments of antibody) chaperon
assisted RNA crystallography to facilitate crystal packing and expediate phase
determination.13
The other popular method to characterize the structure of oligonucleotides is
nuclear magnetic resonance (NMR).14 The advantage of NMR over X-ray
crystallography is that molecules do not have to be crystallized. When a nucleus
which is in a strong constant magnetic field is being perturbed by a weak oscillating
magnetic field, it responds by emitting an electromagnetic signal with a frequency
characteristic of the magnetic field at the nucleus. This happens at the resonance
frequency when the oscillation frequency of the external field matches the intrinsic
frequency of the nuclei. NMR spectra therefore provide information on structures
derived from specific resonance properties of different atomic nuclei in the
samples. Depending on the exact goals of the experiments such as resolution,
different NMR set-ups are used. For instance, two-dimensional nuclear overhauser
effect spectroscopy (NOESY) NMR spectrum can resolve sample structure to a
resolution of five angstrom.
Future outlooks
Oligonucleotides are an important resource for diagnostics and therapeutics, but
in order for them to be clinically useful without adverse side effects, their synthesis
and characterization have to be rigorous. The use of PAGE or LC followed by MS is
a crucial step to separate and purify oligonucleotides. However, the structures of
oligonucleotides also play an important role in their uses such as exploiting their
conformations to mimic viral particles. Therefore, techniques like X-ray
crystallography and NMR are essential to elucidate the molecular structure of
oligonucleotides which will help inform their structure-function relationship, and to
propel the use of molecular design of oligonucleotides to enhance greater use in
biomedical settings. The characterization of oligonucleotides has also focused
largely on genomic DNA, but RNA modifications play important role in physiology
as well. With greater investigations, more RNA modifications and their roles will
also be elucidated.
References:
1. Park JH, Rivière I, Gonen M, et al. Long-Term Follow-up of CD19 CAR
Therapy in Acute Lymphoblastic Leukemia. N Engl J Med. 2018;378(5):449-459.
doi:10.1056/NEJMoa1709919.
2. Veneziano R, Moyer TJ, Stone MB, et al. Role of nanoscale antigen
organization on B-cell activation probed using DNA origami. Nature
Nanotechnology. 2020;15(8):716-723. doi:10.1038/s41565-020-0719-0.
3. Goodchild J. Conjugates of oligonucleotides and modified oligonucleotides:
a review of their synthesis and properties. Bioconjugate Chem. 1990;1(3):165-187.
doi:10.1021/bc00003a001.
4. Cohen AS, Najarian DR, Paulus A, Guttman A, Smith JA, Karger BL. Rapid
separation and purification of oligonucleotides by high-performance capillary gel
electrophoresis. Proc Natl Acad Sci USA. 1988;85(24):9660.
doi:10.1073/pnas.85.24.9660.
5. Zhang Q, Lv H, Wang L, et al. Recent methods for purification and structure
determination of oligonucleotides. Int J Mol Sci. 2016;17(12):2134. Published 2016
Dec 18. doi:10.3390/ijms17122134.
6. Goyon A, Yehl P, Zhang K. Characterization of therapeutic oligonucleotides
by liquid chromatography. Journal of Pharmaceutical and Biomedical Analysis.
2020;182:113105. doi:10.1016/j.jpba.2020.113105.
7. Gilar M, Fountain KJ, Budman Y, et al. Ion-pair reversed-phase highperformance
liquid chromatography analysis of oligonucleotides: retention
prediction. J Chromatogr A. 2002;958(1-2):167-182. doi:10.1016/s0021-
9673(02)00306-0.
8. Pieles U, Zürcher W, Schär M, Moser H. Matrix-assisted laser desorption
ionization time-of-flight mass spectrometry: a powerful tool for the mass and
sequence analysis of natural and modified oligonucleotides. Nucleic Acids
Research. 1993;21(14):3191-3196. doi:10.1093/nar/21.14.3191
9. Kimura S, Dedon PC, Waldor MK. Comparative tRNA sequencing and RNA
mass spectrometry for surveying tRNA modifications. Nature Chemical Biology.
2020;16(9):964-972. doi:10.1038/s41589-020-0558-1.
10. Ho CS, Lam CWK, Chan MHM, et al. Electrospray ionisation mass
spectrometry: principles and clinical applications. Clin Biochem Rev. 2003;24(1):3-
12.
11. Zhang W, Szostak JW, Huang Z. Nucleic acid crystallization and X-ray
crystallography facilitated by single selenium atom. Frontiers of Chemical Science
and Engineering. 2016;10(2):196-202. doi:10.1007/s11705-016-1565-3
12. Mandal PK, Collie GW, Kauffmann B, Huc I. Racemic DNA
crystallography. Angew Chem Int Ed Engl. 2014;53(52):14424-14427.
doi:10.1002/anie.201409014.
13. Sherman E, Archer J, Ye J-D. Fab Chaperone-Assisted RNA Crystallography
(Fab CARC). In: Ennifar E, ed. Nucleic Acid Crystallography: Methods and Protocols.
Springer New York; 2016:77-109. doi:10.1007/978-1-4939-2763-0_7
14. Spring-Connell AM, Evich M, Germann MW. NMR structure determination for
oligonucleotides. Current Protocols in Nucleic Acid Chemistry. 2018;72(1):7.28.1-
7.28.39. doi:10.1002/cpnc.48
©2024 Technology Networks, all rights reserved, Part of the LabX Media Group
Sponsored by
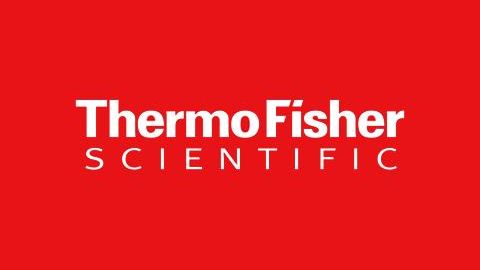
Download the Article for FREE Now!
Information you provide will be shared with the sponsors for this content. Technology Networks or its sponsors may contact you to offer you content or products based on your interest in this topic. You may opt-out at any time.